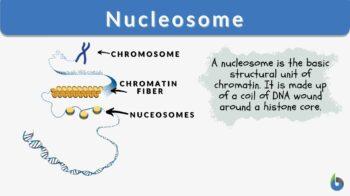
Nucleosome
n., plural: nucleosomes
[ˈnjuːklɪəˌsəʊm]
Definition: basic structural unit of chromatin
Table of Contents
Nucleosome Definition
Every organism is made of deoxyribonucleic acid, also known as DNA. DNA is made up of numerous structural units, which include nucleosomes. What is a nucleosome in biology? To define nucleosome, we must think of what is nucleosome and its function.
A nucleosome is a basic unit of DNA chromatin in eukaryotic organisms. Eukaryotic chromatin is composed of DNA and DNA is present in the cell nucleus. A nucleosome consists of units that assist with the DNA packaging in eukaryotes. The nucleosome is made up of a DNA sequence of roughly 150 base pairs wrapped around a core of histone proteins. The arrangement of the nucleosomes is similar to that of beads on a string. This is the structure of the nucleosome.
The nucleosome diameter is less than that of a human hair and the nucleosome function is to act as a scaffold for the creation of higher-order chromatin structures and a layer of gene-expression regulatory control.
How are nucleosomes formed? Histones serve to wrap chromosomal DNA into tiny nuclei. These are positively charged proteins that bind to negatively charged DNA to form complexes known as nucleosomes. Each nucleosome is made up of 1.65 loops of DNA wrapped around eight histone proteins.
How many nucleosomes are present in a mammalian cell? There are 6.6 x 109 base pairs in mammals so, in a mammalian cell, there are precisely 3.3 x 106 nucleosomes. A typical nucleosome contains how many bp of DNA helix? In eukaryotes, a nucleosome is the fundamental structural unit of DNA wrapping. It is the core particle, which is made up of 146 base pairs (bp) of DNA. Figure 1 shows a nucleosome diagram below.
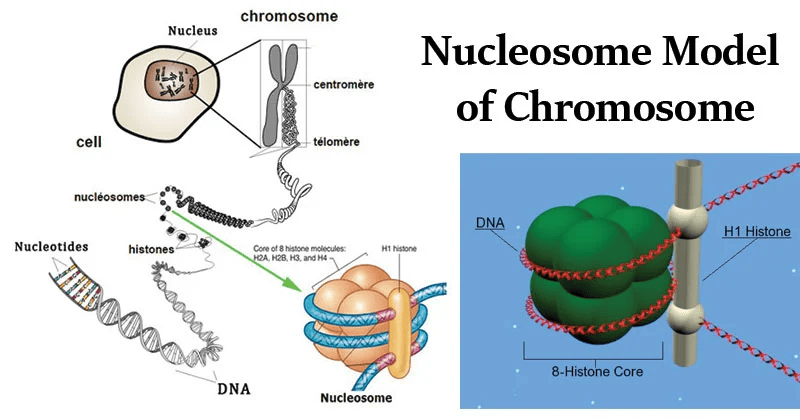
Often nucleosome is confused with a nucleotide. What is the difference between nucleosome and nucleotide? A nucleotide is made up of three parts: a nitrogenous base, a phosphate group, and sugar. A nucleoside is consists of a nitrogenous base and sugar. This is the fundamental distinction between a nucleotide and a nucleoside.
What is the difference between chromatin and nucleosomes? The tight packing of genetic material inside the nucleus is referred to as chromatin and nucleosome. The primary distinction between chromatin and nucleosome is that chromatin refers to the DNA wrapped in histones as a whole, whereas nucleosome refers to the fundamental, repeating structural unit of chromatin.
What is the nucleosome solenoid model? The solenoid model depicts the development of a helical loop in which nucleosomes are stacked one on top of the other in a left-handed pattern around the DNA helix. The linker DNA is gently twisted, forming a long chromatin fiber by linking the nucleosome core with the solenoid or DNA-helix.
A nucleosome is any of the repeating units of the organization of chromatin. It consists of around 200 base pairs and histones. The nucleosome core particle is one in which there is about 146 bp of DNA around the histone octamer. The adjacent nucleosome core particles are joined by a stretch of DNA segment called linker DNA. The linker DNA consists of about 10 to 80 bp. Together, they form a structure reminiscent of a string of beads. Nucleosome formation is essential to pack a large genome into the nucleus. Without histone cores from where the DNA wounds around, the genome would be too lengthy to fit into the nucleus, which roughly has about a diameter of 10 µm. Nucleosomes were first observed by Don and Ada Olins in 1974 and their structure was described by Roger Kornberg.
Synonym: nu body
Structure
The structural composition of the nucleosome is viewed as unique. It encompasses many parts.
Structure of the core particle
Overview
The nucleosome model of the chromosome is a scientific concept that describes how DNA and related proteins are organized in the chromosome. It also clarifies the precise process of DNA folding in the nucleus. Roger Kornberg introduced the concept in 1974, and it is the most widely acknowledged model of chromatin structure, hence the nucleosome model.
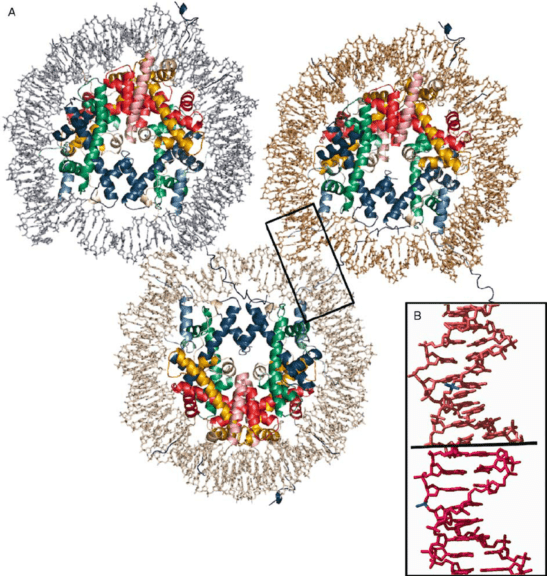
The nucleosome core particle (NCP)
The nucleosome core particle or the nucleosome core is made up of about 146 bp of DNA wrapped in 1.67 left-handed superhelical loops around the histone octamer, which contains two sets of the main histones H2A, H2B, H3, and H4. Adjacent nucleosomes are linked together by a free DNA stretch known as linker DNA (which varies from 10 – 80 bp in length depending on species and tissue type). The whole structure produces a cylinder with a diameter of 11 nm and a height of 5.5 nm.
-
Protein interactions within the nucleosome
The core histone proteins have a distinctive structural pattern known as the “histone fold”, which is composed of three alpha-helices (1-3) separated by two loops (L1-2). Histones in solution form H2A-H2B heterodimers and H3-H4 heterotetramers.
Histones dimerize in an anti-parallel orientation around their long 2 helices, and in the case of H3 and H4, two such dimers form a 4-helix bundle stabilized by significant H3-H3′ contact. Because of interactions between H4 and H2B, which include the development of a hydrophobic cluster, the H2A/H2B dimer attaches to the H3/H4 tetramer.
A core H3/H4 tetramer is sandwiched between two H2A/H2B dimers to produce the histone octamer. The histone octamer is only stable in the presence of DNA or very high salt concentrations due to the very basic charge of all four core histones.
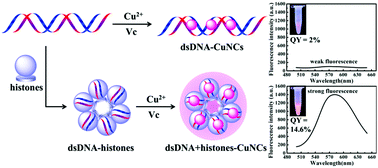
-
Histone – DNA interactions
What are histones and nucleosomes? Histones are basic proteins that supply energy and a structural surface for the DNA that wraps around them. Nucleosomes are the fundamental building blocks of DNA packaging. Do you know if histone proteins are basic or acidic? Histones are proteins that are alkaline.
Histone proteins, DNA segments, and other supporting proteins make up nucleosomes. How many histones are in a nucleosome? The histone nucleosome is the most basic constituent of chromatin. Each nucleosome is made up of around two rounds of DNA wrapped around a group of eight proteins called histones, known as a histone octamer.
Nucleosomes fold up to create a 30 nm fiber nucleosome chromatin, which generates 300-nanometer-long loops. Each histone octamer contains two copies of each histone protein. The different types of histones are H2A, H2B, H3, and H4.
Histone tail domains
Histone tail extensions account for up to 30% of histone mass but are not evident in nucleosome crystal structures due to their high intrinsic flexibility and are assumed to be primarily unstructured. Histones H3 and H2B’s N-terminal tails travel via a channel formed by the minor grooves of the two DNA strands, projecting from the DNA every 20 bp.
The N-terminal tail of histone H4 has a region of very basic amino acids (16-25) that creates a contact with the extremely acidic surface area of another nucleosome’s H2A-H2B dimer in the crystal structure, presumably significant for the higher-order structure of nucleosomes. This interaction is expected to occur under physiological settings as well, implying that acetylation of the H4 tail modifies chromatin’s higher-order structure.
Higher-order structure
The nucleosome’s ability to organize DNA cannot entirely explain the packing of DNA observed in the cell nucleus. Further chromatin compaction into the cell nucleus is required, although the process is not well understood. According to current thinking, repeating nucleosomes with intervening “linker” DNA form a 10-nm-fiber with a packing ratio of five to ten and is described as “beads on a string”. A chain of nucleosomes may be assembled in a 30 nm fiber, a compressed structure with a packing ratio of 50 that requires the presence of the H1 histone to form.
A tetranucleosome crystal structure was presented and used to construct a proposed structure of the 30 nm fiber as a two-start helix. This hypothesis is still subject to debate since it is incompatible with newer electron microscopy results. Beyond that, the structure of chromatin is unknown, but it is commonly assumed that the 30 nm fiber is arranged into loops along a central protein scaffold to form transcriptionally active euchromatin. Compaction results in transcriptionally inactive heterochromatin.
Dynamics
Although the nucleosome is a fairly stable protein-DNA complex, it is not static and has been found to undergo a variety of structural alterations, including nucleosome sliding and DNA site exposure. Nucleosomes can either impede or enhance transcription factor binding depending on the circumstances.
Three significant contributions influence nucleosome positions:
- First, the histone octamer’s intrinsic binding affinity is determined by the DNA sequence.
- Second, through the competitive or cooperative binding of other protein components, the nucleosome might be dislodged or recruited.
- Third, ATP-dependent remodeling complexes may actively translocate the nucleosome.
Nucleosome sliding
When nucleosomes reconstituted onto the 5S DNA positioning sequence were thermally warmed, they were able to move translationally onto neighboring sequences. Later research revealed that this repositioning did not necessitate the breaking of the histone octamer and was consistent with nucleosomes being able to “glide” along with the DNA in cis. CTCF binding sites were discovered to operate as nucleosome positioning anchors in 2008, allowing several flanking nucleosomes to be easily detected when utilized to align diverse genomic signals.
Despite the fact that nucleosomes are fundamentally mobile, eukaryotes have developed a vast family of ATP-dependent chromatin remodeling enzymes to change chromatin structure, many of which do so via nucleosome sliding. Beena Pillai’s research revealed in 2012 that nucleosome sliding is one of the conceivable mechanisms for large-scale tissue-specific gene expression. The findings reveal that the transcription start sites for genes expressed in one tissue are depleted of nucleosomes, but the identical set of genes in another tissue where they are not expressed are nucleosome bound. A diagram of nucleosome sliding can be seen in figure 4 below.
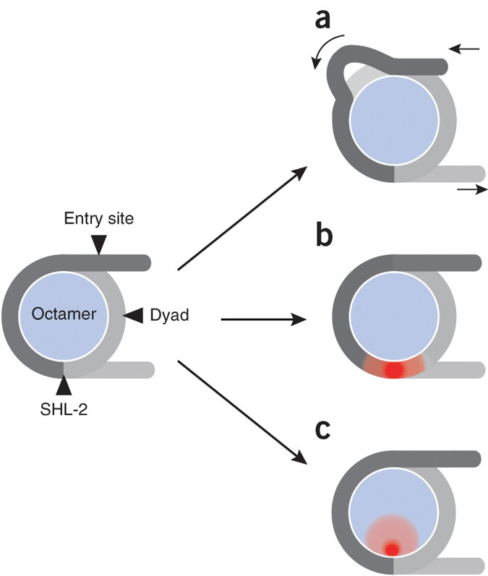
DNA site exposure
The Widom laboratory discovered that nucleosomal DNA is in an equilibrium between wrapped and unwrapped states. Measurements of these rates using time-resolved FRET revealed that DNA within the nucleosome remains fully wrapped for only 250 ms before being unwrapped for 10-50 ms and then rapidly rewrapped.
This implies that DNA does not need to be actively dissociated from the nucleosome, but that there is a significant period of time when it is fully accessible. Indeed, this may be extended to the discovery that inserting a DNA-binding sequence inside the nucleosome enhances the accessibility of surrounding areas of DNA when bound. This proclivity for DNA within the nucleosome to “breathe” has significant functional implications for all DNA-binding proteins that work in the nucleosome.
Nucleosome free region
Active gene promoters have nucleosome free areas (NFR). This permits numerous proteins, such as transcription factors, to access promoter DNA. In S. cerevisae, the nucleosome free region generally extends 200 nucleotides. NFR borders are formed by well-positioned nucleosomes. These nucleosomes are known as the +1-nucleosome and the 1-nucleosome, and they are positioned at conventional distances downstream and upstream of the transcription start site, respectively. The H2A.Z histone variation is also found in the +1-nucleosome and numerous downstream nucleosomes.
Modulating Nucleosome Structure
Eukaryotic genomes are ubiquitously connected with chromatin; nonetheless, cells must control particular loci geographically and temporally independent of bulk chromatin. Cells have developed a number of mechanisms to locally and precisely regulate chromatin structure and function in order to attain the high degree of control necessary to co-ordinate nuclear functions like DNA replication, repair, and transcription. This can include histone covalent modification, histone variant insertion, and non-covalent remodeling by ATP-dependent remodeling enzymes.
Histone post-translational modifications
Histone alterations have been hypothesized to impact transcription since they were identified in the mid-1960s. Because the majority of the early post-translational modifications discovered were localized within the tail extensions that protrude from the nucleosome core, two major ideas on the process of histone modification have emerged. The first theory proposed that they might affect electrostatic interactions between histone tails and DNA in order to “loosen” chromatin structure.
Later, it was postulated that combining these alterations might result in binding epitopes that can be used to attract additional proteins. Given the discovery of additional modifications in the structured sections of histones, it has recently been proposed that these modifications may influence histone-DNA and histone-histone interactions inside the nucleosome core.
Modifications that reduce the charge of the globular histone core (such as acetylation or phosphorylation) are projected to “loosen” core-DNA connection; the degree of the impact is dependent on the position of the change within the core.
Some changes have been linked to gene silencing, whereas others appear to be linked to gene activation. Acetylation, methylation, or ubiquitination of lysine; arginine methylation; and serine phosphorylation are all common alterations. Because this information is not encoded in the DNA but is nevertheless passed down to daughter cells, it is termed epigenetic. The maintenance of a gene’s repressed or activated status is frequently required for cellular differentiation.
Histone variants
Although histones have been shown to be impressively preserved throughout evolution, various alternative forms have been discovered. This variation in histone function is limited to H2A and H3, with H2B and H4 remaining relatively constant. H2A can be replaced by H2AZ (which reduces nucleosome stability) or H2AX (which is associated with DNA repair and T cell differentiation), whereas macroH2A is abundant in inactive X chromosomes in mammals. H3 can be replaced by H3.3 (which connects with activation genes and regulatory elements), and H3 can be replaced by CENPA in centromeres.
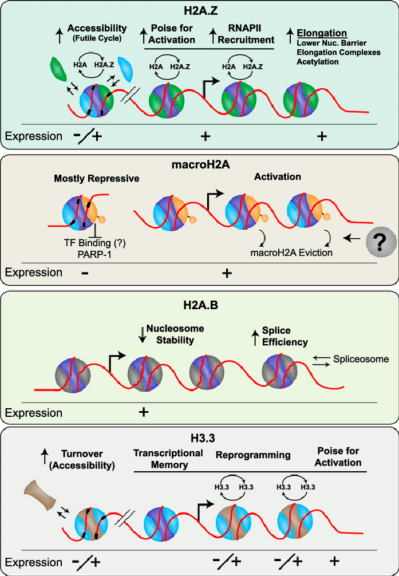
ATP-dependent nucleosome remodeling
The term ATP-dependent chromatin remodeling refers to a variety of different events. Remodeling enzymes have been demonstrated to move nucleosomes along DNA, break histone-DNA interactions to the point of destabilizing the H2A/H2B dimer, and induce negative superhelical torsion in DNA and chromatin.
Swr1 remodeling enzyme has recently been found to insert the variant histone H2A.Z into nucleosomes. At the moment, it is unclear whether all of these are distinct reactions or simply alternative outcomes of a common mechanism. What they all have in common, and indeed what distinguishes ATP-dependent chromatin remodeling, is that they all result in altered DNA accessibility.
Studies of gene activation in vivo and remodeling in vitro have indicated that chromatin remodeling processes and transcription-factor binding are cyclical and periodic in nature. While the implications for the response mechanism of chromatin remodeling are unknown, the system’s dynamic character may allow it to adapt to external stimuli more quickly. According to a recent study, nucleosome locations fluctuate dramatically throughout the development of mouse embryonic stem cells, and these changes are connected to the binding of developmental transcription factors.
Dynamic Nucleosome Remodeling Across the Yeast Genome
In 2007, researchers cataloged nucleosome sites in yeast and discovered that nucleosomes are reduced in promoter regions and replication origins. Nucleosomes appear to encompass around 80% of the yeast genome, and the pattern of nucleosome placement is clearly related to DNA regions that control transcription, areas that are transcribed, and sites that begin DNA replication.
Recently, a new study investigated dynamic changes in nucleosome repositioning during a global transcriptional reprogramming event in yeast to determine the impact on nucleosome displacement during genome-wide transcriptional alterations (Saccharomyces cerevisiae). The findings indicated that nucleosomes located to promoter regions are shifted in response to stress (like heat shock).
Furthermore, nucleosome removal usually corresponded to transcriptional activation, while nucleosome replacement usually corresponded to transcriptional repression, presumably because transcription factor binding sites became more or less accessible, respectively. To effect these transcriptional changes, only one or two nucleosomes were repositioned at the promoter.
However, nucleosome repositioning was observed even in chromosomal regions that were not associated with transcriptional changes, implying that the covering and uncovering of transcriptional DNA do not always result in a transcriptional event. After transcription, the rDNA region must be protected from damage, and it has been suggested that HMGB proteins play a significant role in protecting the nucleosome-free region.
Nucleosome Assembly In Vitro
Purified natural or recombinant nucleosomes histones can be used to build nucleosomes in vitro. The use of salt dialysis is a common method for loading the DNA around the histones. At a salt concentration of 2 M, the histone octamers and a bare DNA template can be incubated together. As the salt concentration is gradually reduced, the DNA will equilibrate to a position where it is wrapped around the histone octamers, forming nucleosomes. This reconstitution technique, under the right circumstances, allows the nucleosome positioning affinity of a particular sequence to be empirically mapped.
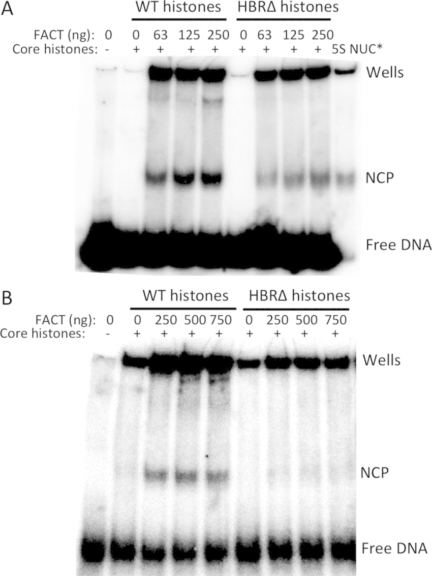
Disulfide cross-linked nucleosome core particles
A recent breakthrough in the creation of nucleosome core particles with increased stability involves the use of site-specific disulfide crosslinks. In the nucleosome core particle, two distinct crosslinks can be inserted. The first one uses an inserted cysteine (N38C) to crosslink the two copies of H2A, resulting in a histone octamer that is stable against H2A/H2B dimer loss during nucleosome reconstruction.
A second crosslink can be formed by including a convertible nucleotide between the H3 N-terminal histone tail and the nucleosome DNA ends. At low particle concentrations and high salt concentrations, the DNA-histone octamer crosslink stabilizes the nucleosome core particle against DNA dissociation.
Nucleosome Assembly In Vivo
Nucleosomes are the fundamental DNA packing units composed of histone proteins around, which DNA is wrapped. They function as a scaffold for the creation of higher-order chromatin structure as well as a layer of regulatory control over gene expression. Behind the replication fork, nucleosomes are rapidly assembled onto freshly produced DNA.
H3 and H4
Histones H3 and H4 from disintegrated old nucleosomes are preserved nearby and dispersed at random on the freshly produced DNA. They are put together by the chromatin assembly factor-1 (CAF-1) complex, which is made up of three subunits (p150, p60, and p48). The replication coupling assembly factor assembles newly produced H3 and H4 (RCAF). Asf1, a subunit of RCAF, interacts with freshly produced H3 and H4 proteins. The ancient H3 and H4 proteins maintain their chemical changes, which adds to the epigenetic signature’s transmission. As part of the chromatin maturation process, freshly generated H3 and H4 proteins are progressively acetylated at distinct lysine sites.
H2A and H2B
Unlike old H3 and H4, old H2A and H2B histone proteins are released and destroyed, allowing freshly formed H2A and H2B proteins to be integrated into new nucleosomes. H2A and H2B combine to form dimers that are then placed onto nucleosomes by the nucleosome assembly protein-1 (NAP-1) which also aids in nucleosome sliding. ATP-dependent nucleosome-remodeling complexes comprising enzymes such as Isw1, Ino80, and Chd1 space the nucleosomes before they are assembled into a higher-order structure.
Recent Developments
Circulating nucleosomes
Global dysregulation of epigenetic markers on circulating nucleosomes, such as DNA methylation and histone alterations, has also been linked to colorectal and pancreatic cancer. Changes in histone modification patterns detected on circulating nucleosomes may thus be effective blood-based biomarkers for early cancer detection.
Fragile nucleosomes
The Fragile Nucleosome Journal Club is a new Fragile Nucleosome community effort in which students and postdocs interested in chromatin and gene control may actively debate current works with peers from all across the world. The Fragile Nucleosome Journal Club follows a different format from the Fragile Nucleosome Seminars. Participants will debate a preprint or recent work with the assistance of an invited expert in the subject.
Try to answer the quiz below to check what you have learned so far about nucleosomes.
References
- A Typical Nucleosome Contains ______.(A) 100bp Of DNA Helix.(B) 200bp Of DNA Helix.(C) 300bp Of DNA Helix.(D) 400bp Of DNA Helix. – Biology Q&A. (n.d.). BYJU’S. Retrieved March 7, 2022, from https://byjus.com/questions/a-typical-nucleosome-contains-a-100bp-of-dna-helix-b-200bp-of-dna-helix-c-300bp-of-dna-helix-d-400bp-of-dna-helix/
- Allfrey, V. G., Faulkner, R., & Mirsky, A. E. (1964). ACETYLATION AND METHYLATION OF HISTONES AND THEIR POSSIBLE ROLE IN THE REGULATION OF RNA SYNTHESIS. Proceedings of the National Academy of Sciences of the United States of America, 51, 786–794. https://doi.org/10.1073/pnas.51.5.786
- Aryal, S. (2022, February 24). Chromosomes- Definition, Structure, Types, Model, Functions. Microbe Notes. https://microbenotes.com/chromosomes/
- Cosgrove, M. S., Boeke, J. D., & Wolberger, C. (2004). Regulated nucleosome mobility and the histone code. Nature Structural & Molecular Biology, 11(11), 1037–1043. https://doi.org/10.1038/nsmb851
- Difference Between Histones and Nucleosomes | Compare the Difference Between Similar Terms. (n.d.-a). Retrieved March 7, 2022, from https://www.differencebetween.com/difference-between-histones-and-vs-nucleosomes/
- Difference Between Histones and Nucleosomes | Compare the Difference Between Similar Terms. (n.d.-b). Retrieved March 7, 2022, from https://www.differencebetween.com/difference-between-histones-and-vs-nucleosomes/
- DNA Packaging: Nucleosomes and Chromatin | Learn Science at Scitable. (n.d.). Retrieved March 7, 2022, from http://www.nature.com/scitable/topicpage/dna-packaging-nucleosomes-and-chromatin-310
- DNA Packaging: Nucleosomes and Chromatin | Learn Science at Scitable. (n.d.). Retrieved March 7, 2022, from https://www.nature.com/scitable/topicpage/dna-packaging-nucleosomes-and-chromatin-310/
- Dyer, P. N., Edayathumangalam, R. S., White, C. L., Bao, Y., Chakravarthy, S., Muthurajan, U. M., & Luger, K. (2004). Reconstitution of nucleosome core particles from recombinant histones and DNA. Methods in Enzymology, 375, 23–44. https://doi.org/10.1016/s0076-6879(03)75002-2
- Fenley, A. T., Adams, D. A., & Onufriev, A. V. (2010). Charge state of the globular histone core controls stability of the nucleosome. Biophysical Journal, 99(5), 1577–1585. https://doi.org/10.1016/j.bpj.2010.06.046
- Fragile Nucleosome Journal Club – Gene Regulation – Teif Lab. (n.d.). Retrieved March 7, 2022, from https://generegulation.org/fragile-nucleosome/fn-journal-club/
- Frouws, T. D., Barth, P. D., & Richmond, T. J. (2018). Site-Specific Disulfide Crosslinked Nucleosomes with Enhanced Stability. Journal of Molecular Biology, 430(1), 45–57. https://doi.org/10.1016/j.jmb.2017.10.029
- Havas, K., Flaus, A., Phelan, M., Kingston, R., Wade, P. A., Lilley, D. M., & Owen-Hughes, T. (2000). Generation of superhelical torsion by ATP-dependent chromatin remodeling activities. Cell, 103(7), 1133–1142. https://doi.org/10.1016/s0092-8674(00)00215-4
- Lakna. (2018, February 14). Difference Between Chromatin and Nucleosome | Definition, Structure, Role, Similarities and Differences. Pediaa.Com. https://pediaa.com/difference-between-chromatin-and-nucleosome/
- Li, B., Carey, M., & Workman, J. L. (2007). The role of chromatin during transcription. Cell, 128(4), 707–719. https://doi.org/10.1016/j.cell.2007.01.015
- Murugesapillai, D., McCauley, M. J., Maher, L. J., & Williams, M. C. (2017). Single-molecule studies of high-mobility group B architectural DNA bending proteins. Biophysical Reviews, 9(1), 17–40. https://doi.org/10.1007/s12551-016-0236-4
- Nagaich, A. K., Walker, D. A., Wolford, R., & Hager, G. L. (2004). Rapid periodic binding and displacement of the glucocorticoid receptor during chromatin remodeling. Molecular Cell, 14(2), 163–174. https://doi.org/10.1016/s1097-2765(04)00178-9
- Nucleosome. (n.d.-b). Genome.Gov. Retrieved March 7, 2022, from https://www.genome.gov/genetics-glossary/Nucleosome
- Nucleosome assembly protein 1 exchanges histone H2A-H2B dimers and assists nucleosome sliding—PubMed. (n.d.). Retrieved March 7, 2022, from https://pubmed.ncbi.nlm.nih.gov/15516689/
- Shivaswamy, S., Bhinge, A., Zhao, Y., Jones, S., Hirst, M., & Iyer, V. R. (2008). Dynamic remodeling of individual nucleosomes across a eukaryotic genome in response to transcriptional perturbation. PLoS Biology, 6(3), e65. https://doi.org/10.1371/journal.pbio.0060065
- Tyler, J., Adams, C., Chen, S.-R., Kobayashi, R., Kamakaka, R., & Kadonaga, J. T. (1999). The RCAF complex mediates chromatin assembly during DNA replication and repair. Nature. https://doi.org/10.1038/990147
- Yamasu, K., & Senshu, T. (1990). Conservative segregation of tetrameric units of H3 and H4 histones during nucleosome replication. Journal of Biochemistry, 107(1), 15–20. https://doi.org/10.1093/oxfordjournals.jbchem.a122999
©BiologyOnline.com. Content provided and moderated by Biology Online Editors.