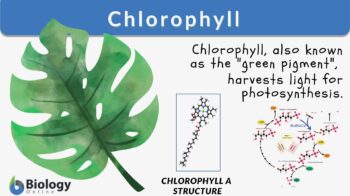
Chlorophyll
n., plural: chlorophylls
[kloʁoˈfʏl]
Definition: green pigment found in the chloroplasts
Table of Contents
Why are most plants green? Have you ever had the same question? Perhaps, you’ve been told that the plants are green because of their green pigment. But have you wondered, of all the colors, why green? Before we try to figure out the answer to that, let’s define pigment. In essence, a pigment means a coloring material; it is a dye, a paint. But in biology, a pigment, by definition, refers to a colored substance naturally produced by an organism. For instance, our skin, although may differ from one person to another, generally contains a special pigment called melanin. This pigment is produced by special cells in our skin called melanocytes. Melanin is a pigment not just found in our skin. It is also produced and responsible for the color of certain animal fur, feathers, and other animal body parts. In fact, it is also even present in plants and is responsible for the brown and black color of certain seeds. (Ref. 1: Glagoleva et al., 2020) Having understood what a pigment is, let us now learn more about another pigment that renders many plants green, chlorophyll.
Chlorophyll Definition and History
Chlorophyll is defined as the green pigment found in plants. French pharmacists, Joseph Bienaimé Caventou and Pierre Joseph Pelletier, coined the term “chlorophyll” in 1817.
Definition of chlorophyll: “Nous n’avons aucun droit pour nommer une substance connue depuis long-temps, et à l’histoire de laquelle nous n’avons ajouté que quelques faits ; cependant nous proposerons, sans y mettre aucune importance, le nom de chlorophyle, de chloros, couleur, et φυλλον, feuille : ce nom indiquerait le rôle qu’elle joue dans la nature.” (We have no right to name a substance [that has been] known for a long time, and to whose story we have added only a few facts ; however, we will propose, without giving it any importance, the name chlorophyll, from “chloros”, “color”, and φυλλον, “leaf”: this name would indicate the role that it plays in nature.)” – Caventou, J.B. and Pelletier, P.J. (1906) (Ref.2)
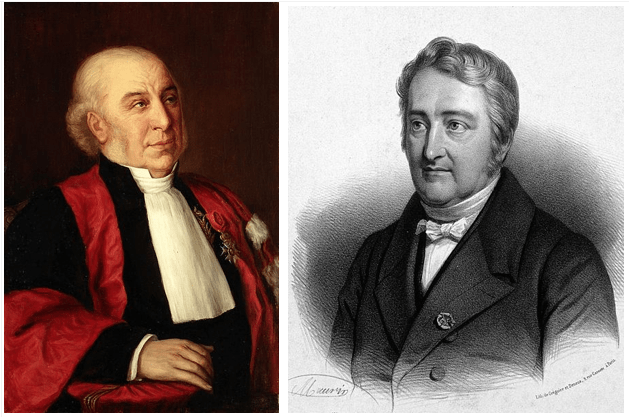
Chlorophyll is the green pigment found in the chloroplasts of higher plants and in the cells of photosynthetic microorganisms (e.g. cyanobacteria), which is primarily involved in absorbing light energy for photosynthesis. Etymology: Greek “chloros”, meaning “green” and “phyllon”, meaning “leaf”. Synonym: green pigment. Compare: carotenoid
Chlorophyll Structure
The chlorophyll molecule is a chlorin pigment with magnesium at the center of the chlorin ring. Refer to Figures 2- below. The magnesium is represented by the color green at the center of the ball-and-stick model of the chlorophyll molecules shown.
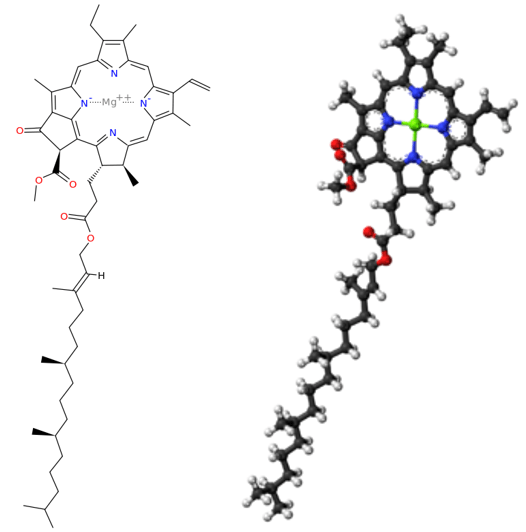
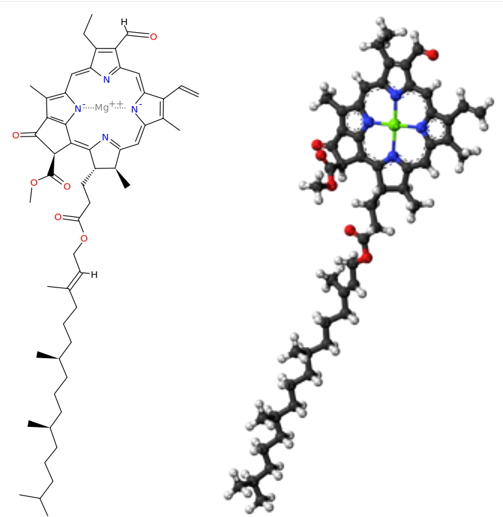
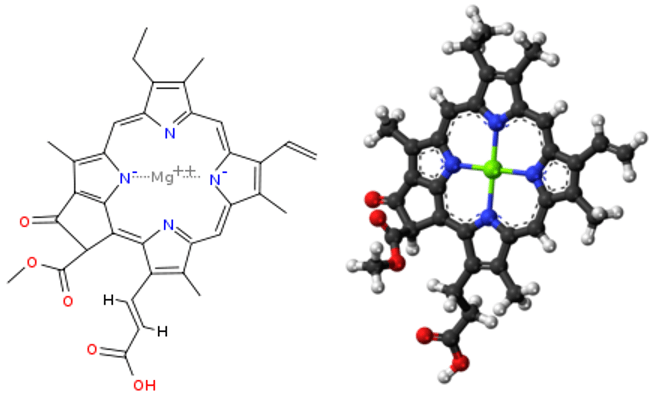
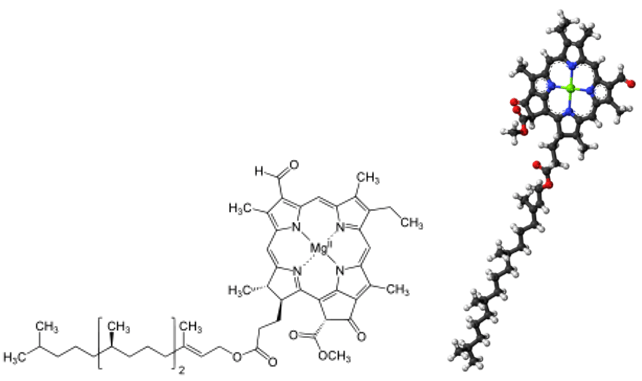
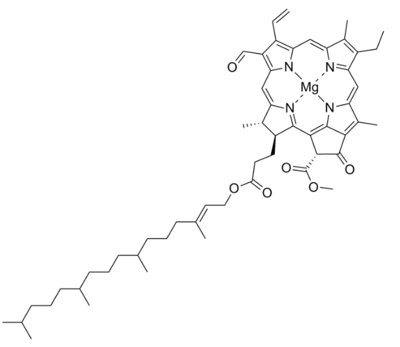
Figure 6: Chlorophyll f (structure). Left: chemical structure. Credit: David Richfield, public domain.
Chlorin ring
As shown in the Figures above, the chemical structures vary among the different types of chlorophyll molecules. They have one thing in common in terms of structural features; all of them have a chlorin ring, with magnesium at the center. Not to be confused with the chemical element chlorine (symbol: Cl, atomic number: 17), chlorins are cyclic tetrapyrroles similar to the heme of the hemoglobin (i.e., chlorins are to chlorophyll as heme to the hemoglobin). One major difference between chlorins and heme, though, is that magnesium (Mg) is the core metal atom whereas in heme it is iron (Fe).
Sidechains
Sidechains are bound to the chlorin ring and this is one of the reasons that characterize a particular type of chlorophyll. The absorption spectrum of light of the chlorophyll molecule varies depending on the side chain attached to the chlorin ring. (Ref.3: Lange et al., 1981)
The chlorin ring with the specific sidechain bound to it is the chromophore of the chlorophyll molecule, meaning it is the part of the molecule that is responsible for its color.
Hydrocarbon tail
Some have a long hydrocarbon tail that anchors the molecule to certain proteins, particularly the hydrophobic proteins in the thylakoid of chloroplast (thylakoid and chloroplast will be discussed in this section).
Types of Chlorophyll
There are about five closely related chlorophylls:
- chlorophyll a
- chlorophyll b
- chlorophyll c
- chlorophyll d
- chlorophyll e
- chlorophyll f
All of them reflect the green light. However, there are slight differences. For instance, they differ slightly in their structure causing them to appear in different shades of green. Chlorophyll a, in particular, is a blue-green pigment whereas chlorophyll b is a yellow-green pigment. See Table 1 for further differences of the different types of chlorophyll.
Table 1: Types of Chlorophylls |
|||
---|---|---|---|
Types | Features | Best Wavelength Spectrum Absorption | Examples |
Chlorophyll a | The main light-harvesting pigment present in all photosynthetic organisms
Molecular formula: C55H72O5N4Mg |
400-450 nm and 650-700 nm | higher plants, red algae, green algae |
Chlorophyll b | It functions as a light-harvesting pigment that passes on the light excitation to chlorophyll a.
Molecular formula: C55H70O6N4Mg |
450-500 nm and 600-650 nm | higher plants, green algae |
Chlorophyll c | An accessory pigment found in certain marine algae
Molecular formula: C35H28O5N4Mg |
447–452 nm | diatoms, dinoflagellates, brown algae |
Chlorophyll d | The form of chlorophyll found in photosynthetic organisms thriving in moderately deep water
Molecular formula: C54H70O6N4Mg |
Infrared light | red algae, cyanobacteria (blue-green algae) |
Chlorophyll e | An accessory pigment and a rare type, isolated from very few algal species, such as in some golden algae. No sufficient information is available about this type of chlorophyll, including the chemical structure and molecular formula. | [no data] | Tribonema bombycinum and Vaucheria hamata |
Chlorophyll f | The form of chlorophyll in aquatic organisms that enable the absorption of near-infrared light
Molecular formula: C55H70O6N4Mg |
Near-infrared light | Cyanobacteria |
Chlorophyll – Green Pigment
Having learned the structure and types of chlorophyll, let us now answer our previous questions. Why are plants green? We have always heard that the green plant has such a color because of the chlorophyll pigment, which is, essentially, referred to as the green pigment. But why is chlorophyll green? Why not violet? Or blue? Or black? Plants are perceived as green because the chlorophyll pigments do not absorb well the green wavelength of the visible light.
The chlorophyll pigments do absorb well blue and red light but not a green light. The green light is reflected and then reaches our eyes and so we perceive them as green. The presence of plant structures like cell walls that are rich in cellulose seems to reflect the green light and may have hindered chlorophyll to absorb the green light very well. (Ref.4: Virtanen, et al., 2020)
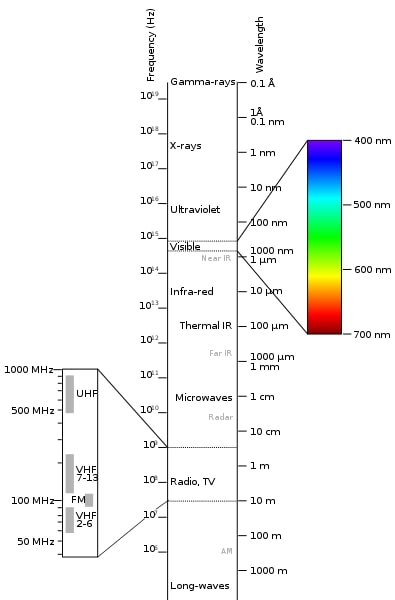
How humans perceive “greenness”
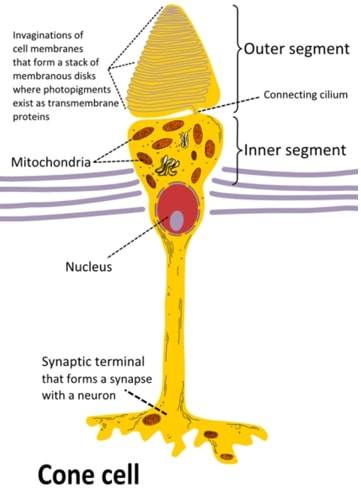
The cone cells (or cones) are photoreceptor cells in the retinas of our eyes that work better at day time (when there is bright light) as opposed to the rod cells (or rods), which are another type of photoreceptors that work better at night time (when there is dim light). While rods are for night vision and peripheral vision, the cones are primarily responsible for color perception (or color vision). Our perception of the green color is attributed to our M-cones (medium-type) that are stimulated by the light at peak wavelength at approximately 534-545 nm, in the green region of the spectrum. The other two types of cones are L-cones (long-type), which respond the most to longer wavelengths of the visible light (i.e. peak wavelength at ~564-580 nm, which is in the yellow-green region), and S-cones (short-type), which respond the most to shorter wavelengths (peak wavelength at ~420-440 nm, which is in the blue region). (Hunt, R. W. G., 2004 Ref.5) All cone types have varying photopsin proteins in which their optimum wavelength absorption depends on their structural conformation. Three types of opsins: (1) long-wavelength-sensitive (OPN1LW) opsin (yellow-green sensitive opsin, (2) medium-wavelength-sensitive opsin (green-sensitive opsin), and (3) short-wavelength-sensitive opsin (blue-sensitive opsin).
Chlorophyll Functions
What does chlorophyll do? The chlorophyll best absorbs light in the blue and red regions of the visible light. It is found in the thylakoid membrane system of a photosynthetic cell.
Thylakoids: location and structure
In eukaryotes, such as plants, the thylakoids are found inside the chloroplast. The chloroplast is an organelle whose thylakoids appear as stacks or piles of coins (collectively called a granum) interconnected by a lamella.
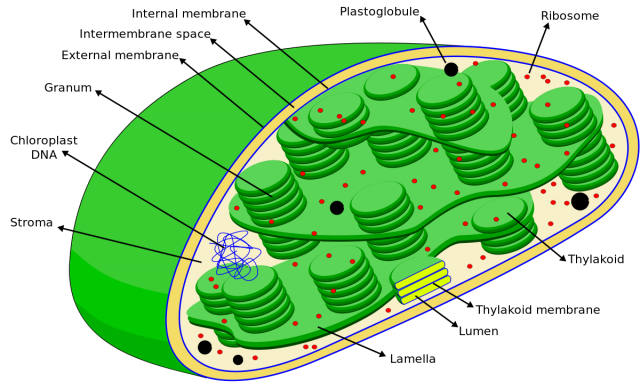
In photosynthetic prokaryotes, such as cyanobacteria, green sulfur bacteria, purple bacteria, heliobacteria, and Chloroflexi bacteria, the thylakoids appear as infoldings of the plasma membrane. These infoldings are where chlorophyll (for cyanobacteria) and chlorophyll-related pigment (bacteriochlorophyll, in other photosynthetic bacteria) attach to and so serve as the site of photosynthesis.
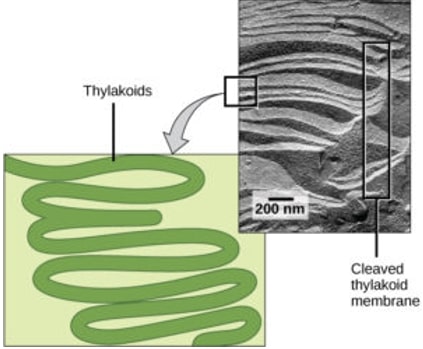
The thylakoids have two main parts: (1) the thylakoid membrane where various membrane proteins are embedded (e.g. Photosystem II) and (2) the thylakoid lumen that contains various lumenal proteins.
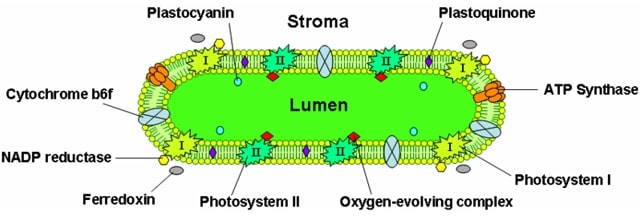
The thylakoid membrane contains various integral proteins that form protein complexes for light harvesting. They are Photosystem I, Photosystem II, cytochrome b6f complex, and ATP synthase. Because the thylakoid membrane has these proteins, it serves as the site for the light-dependent reactions of photosynthesis.
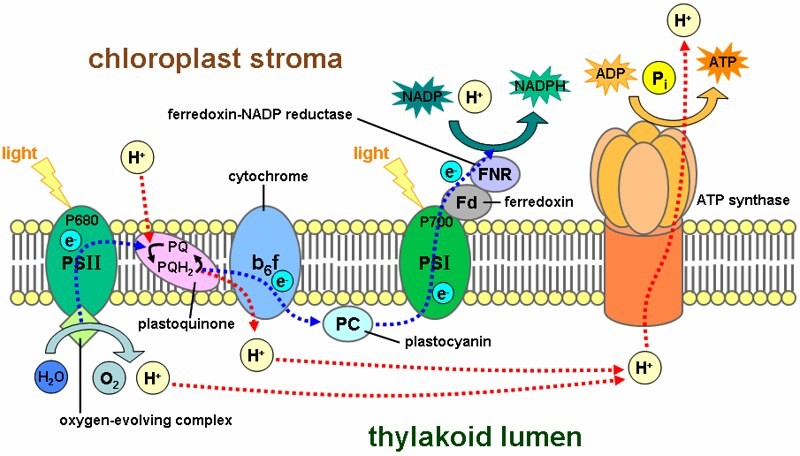
The thylakoid lumen contains lumenal proteins. For example, plastoquinone (PQ) and plastocyanin (PC) are lumenal proteins that are involved in the electron transport chain. Unlike plastocyanin that moves juxtapose the thylakoid membrane, the plastoquinone can incorporate itself into the thylakoid membrane during electron transfer.
Chloroplast stroma
The stroma is the matrix of the chloroplast. It is the fluid surrounding the thylakoids. It is where the light-independent reactions (dark reactions) of photosynthesis take place. In Figure 11, a stromal protein that is part of the electron transport chain is the ferredoxin (Fd), which in this diagram, shuttles the electrons to the NADP to form NADPH, through the help of the enzyme, ferredoxin-NADP reductase.
Photosystems: chlorophyll in photosynthesis
For land plants, the main chlorophyll pigments are chlorophyll a and b. Chlorophyll a, however, is the type of chlorophyll that can convert light energy and thus participates directly in the light reaction of photosynthesis. Chlorophyll b, in contrast, can absorb light energy but eventually relays the energy to chlorophyll a. Because of this, chlorophyll a is the most predominant form of chlorophyll pigment and explains why it is depicted as universal, meaning it is present in all photosynthetic organisms. Nevertheless, chlorophyll b is essential in boosting the efficacy of photosynthesis.
If you will remember, different chlorophylls have different light absorption efficacies. Chlorophyll a absorbs the red color of light. Chlorophyll a is best at absorbing photons at 400-450 nm and 650-700 nm whereas chlorophyll b is best at 450-500 nm and 600-650 nm (see Figure 12 for chlorophyll absorption spectrum).
And so, the presence of chlorophyll b and other accessory pigments (e.g., carotenoids) further improves light gathering or absorption capacity. This is particularly important for shade plants, i.e., plants that grow below the tall trees. The presence of more chlorophyll b than chlorophyll a helps them collect light better despite growing under taller trees. (Ref.6: Lange et al., 1981) The same advantage applies to plants with abundant carotenoids. These accessory pigments enable them to absorb certain wavelengths not efficiently absorbed by the chlorophyll pigments.
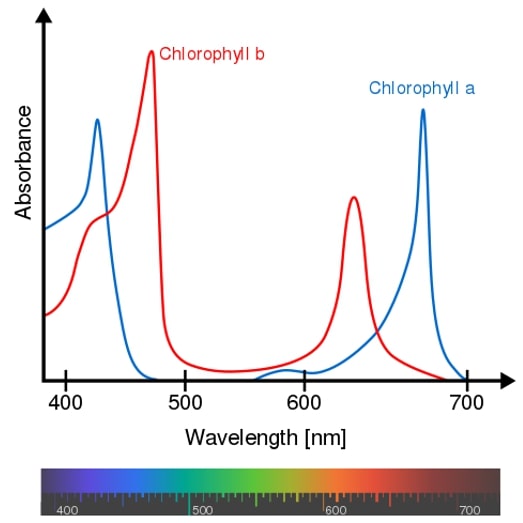
So, how does chlorophyll work? The chlorophyll, together with the accessory pigments, make up a photosystem. A photosystem is a complex in the thylakoid that is responsible for capturing light and causing a series of redox (oxidation-reduction) reactions. In other words, the photosystem is where the light reactions take place.
There are two photosystems: photosystem I (PS I) and photosystem II (PS II). (see Figure 11) The two photosystems differ essentially in their reaction centers. The reaction center of Photosystem I is P700 whereas the reaction center of Photosystem II is P680. P700 is the reaction center that is most reactive and best in absorbing at 700nm (far-red light). P680 is the reaction center that is best at absorbing light at 680nm (red light). Apart from that, Photosystem II is also responsible for the ‘splitting of water components’ during the photosynthesis process. (see Figure 11)
As described above, the primary role of chlorophyll and other pigments is to harvest light, particularly photons. When a photon of light is absorbed by a pigment, this leads to the pigment’s excitation.
In an excited state, the molecule has to give off its electron to return to its original “ground” state. The electron is transferred to the next pigment, and this leads to its excitation. This leads to a series of excitation and returning to the ground state until it reaches chlorophyll a, which resides in the reaction center of a photosystem.
Another molecule resides in the reaction center that serves as the primary electron acceptor. It accepts two electrons from the excited chlorophyll a. The primary acceptor, then, transfers the two electrons to the electron transport chain.
Having lost the electrons to the electron transport chain, the electrons in the reaction center are “restored” by receiving the electrons from the splitting of water (H2O). With the splitting of water, oxygen (O2) is released as a photosynthetic byproduct. Again, take note that the splitting of water and the concomitant release of O2 involves Photosystem II and not Photosystem I. (see Figure 11)
So how about the electrons of the excited primary acceptor in the reaction center of the Photosystem I following the absorption of light? It also loses two electrons when it relays them to ferredoxin. Then, it is filled again by the electrons from the electron transport chain. See Figure 11.
Noncyclic and cyclic electron flow
As depicted in Figure 11, the electrons flow is noncyclic, meaning the electrons do not cycle back to the photosystem from where they came. This is in contrast to another type of electron flow, which is cyclic, meaning the electrons cycle back to the photosystem. See Figure 13.
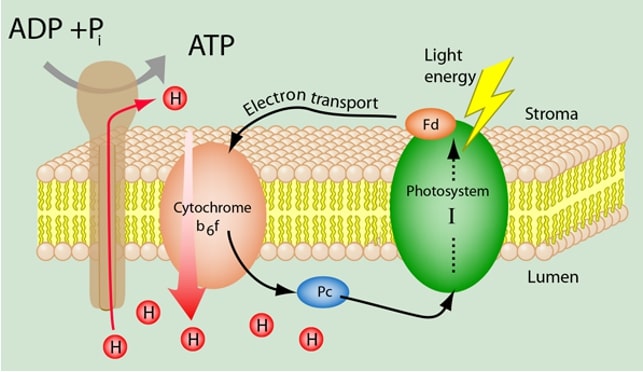
What are other differences between the noncyclic and the cyclic electron flow? In the noncyclic electron flow, NADPH is formed whereas in the cyclic electron flow it is not. Instead of shuttling the electrons to the NADP, the ferredoxin sends them back to the cytochrome b6f, to plastocyanin, and eventually back to Photosystem I. What else? There is also no oxygen released as water is not split in the process.
NADPH is an energy-carrying molecule and a potent reducing agent. Similar to ATP the NADPH stores chemical energy, which is used when making glucose and other organic compounds. Calvin Cycle, in particular, is a pathway wherein CO2 is made into sugar, and ATP and NADPH supply the energy.
In summary, for every 3 CO2 molecules, 9 ATP molecules and 6NADPH are utilized to produce 1 glyceraldehyde-3-phosphate (a sugar).
The noncyclic electron flow occurs more often than the cyclic electron flow so that ATP and NADPH can support the process of making sugar. However, because more ATP molecules are used than NADPH in the Calvin Cycle, the surplus of NADPH is prevented by “pushing” the electrons through the electron transport chain to make more ATP (than make NADPH) to meet the ATP demand in the Calvin Cycle.
Overall, the chlorophyll and the other pigments in the light-harvesting unit (Photosystem) harness the light energy from the sun (or any light source) to convert it into chemical energy (ATP and NADPH) that can sustain the process of making sugars.
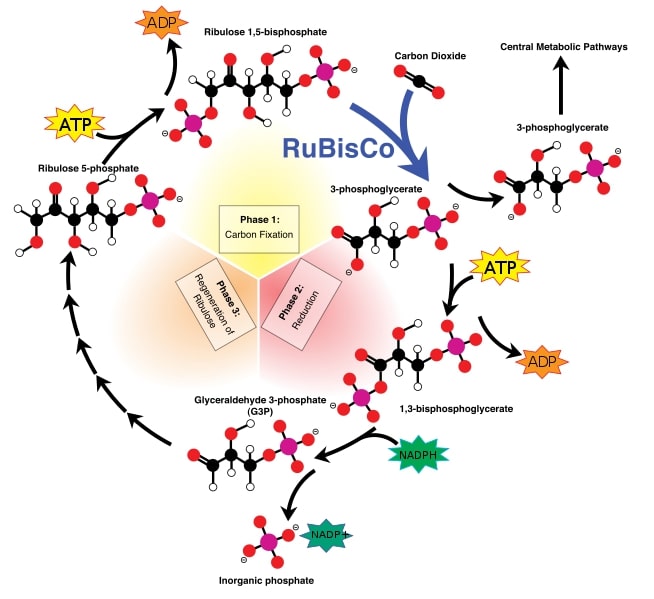
For the process of photosynthesis: Plant Metabolism – Photosynthesis Tutorial
Chlorophyll Benefits
The chlorophyll pigment is certainly one of the most essential natural pigments on Earth. Without them, autotrophs, like plants, will lose their ability to make their own food efficiently. We all know plants play a vital role in the ecosystem as they take the role of producers. They deserve to be called the “producers” because they are the ones producing their own food by utilizing inorganic compounds in the presence of light through a process called photosynthesis. We, on the contrary, eat others to survive. Because we aren’t producers and we rather consume other organisms for food, we are referred to as consumers. Based on the types of nutrition, plants are also referred to as autotrophs whereas consumers, heterotrophs.
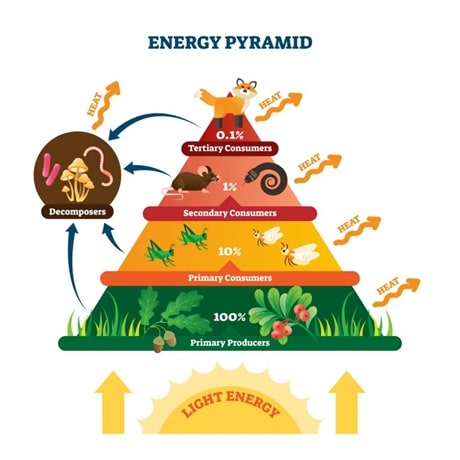
Apart from the ecological importance, the benefits of chlorophyll may extend to human health and wellness. Semi-synthetic derivatives of chlorophyll (liquid chlorophyll or chlorophyll water) are created for use as alternative medicine. Sodium/copper chlorophyllin is one example of a chlorophyll derivative that is taken as a common dietary supplement (and a food colorant). Some of its uses are as follows:
- Tissue repair: chlorophyll is used to enhance wound healing and reduce inflammation. It seems to speed up healing as it can reduce bacterial growth.
- Natural deodorant: chlorophyll is also used in helping neutralize the odor of bad breath, urine, or feces. So, this is used by people who have fecal incontinence or who have had a colostomy.
- Detoxification: it is claimed to have detoxifying effects and beneficial effects against certain cancers.
There have been mixed results from different independent studies on the effectiveness of therapeutic chlorophyll. Thus, more studies are still being conducted to provide definitive proof of chlorophyll efficacy for such uses.
Chlorophyll Examples
Plants
In plants, chlorophyll is the major photosynthetic pigment. The chloroplast contains a great number of chlorophyll pigments to absorb light energy. Some plants grow a shoot that is green throughout. Examples are herbs that have abundant chlorophyll pigments not just in leaves but also on stems. Conversely, there are plants that eventually develop into thick brownish stems called bark. Nevertheless, their leaves remain green and serve as the main plant organ for photosynthesis. While some trees remain “evergreen” by bearing leaves throughout the season there are also trees that lose their leaves at the end of the growing season. They are referred to as “deciduous”. Deciduous trees break down their chlorophylls, leaving their leaves to take a different color, such as yellow, purple, red, or brown. They tend to take the nutrients from the leaves to the stems and roots as an adaptation to the winter or dry season. The leaves are shed. Then, by the end of the winter or dry season, they regrow their leaves rich in chlorophylls again to collect light for photosynthesis.
Algae
In algae, chlorophylls are also found inside the chloroplasts. Most algae are capable of photosynthesis. A few algal species lack pigments and therefore they are colorless and heterotrophic. Photosynthetic algae are a major biological source of atmospheric oxygen. They are a diverse group of microscopic and macroscopic autotrophs that are commonly thriving in various bodies of water. While most of them are aquatic, some are found in terrestrial habitats, such as moist soil, trees, and rocks. Others live in lichens where they form symbiotic relationships with certain fungal species.
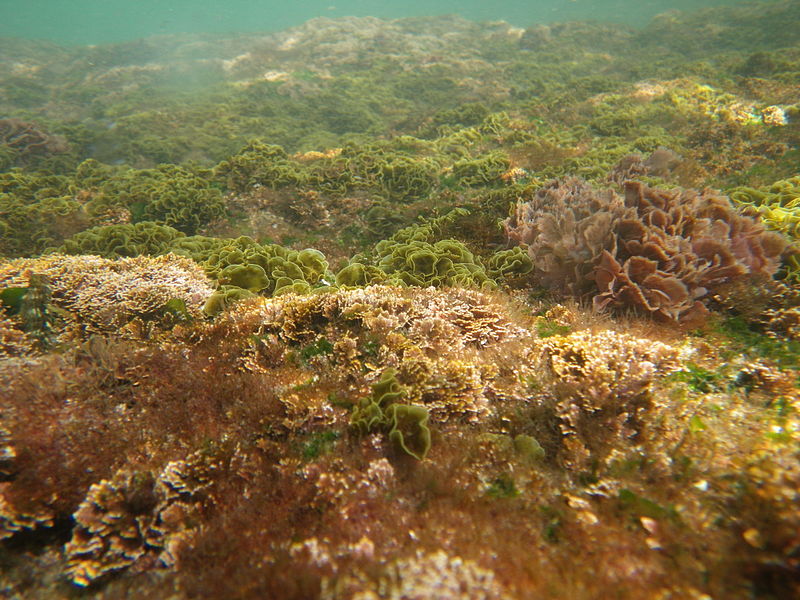
Cyanobacteria
Cyanobacteria are examples of prokaryotes that are photosynthetic because of the presence of chlorophyll. Other photosynthetic bacteria, such as green sulfur bacteria, purple bacteria, Chlroflexi, and heliobacteria do not possess chlorophyll but only a similar photosynthetic pigment called bacteriochlorophyll. Bacteriochlorophyll is not involved in forming oxygen as a byproduct during photosynthesis. Chlorophyll does that, particularly chlorophyll a.
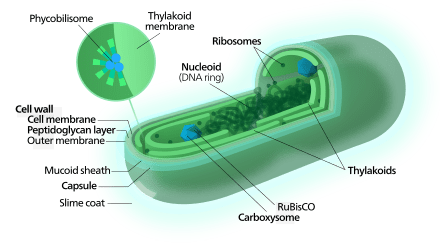
Cyanobacteria have thylakoid membranes but are not organized inside an organelle. Rather, the thylakoids are infoldings of the plasma membrane of the cyanobacterial cell. Unlike plant thylakoids that are organized into stacks or discs (granum), the cyanobacterial thylakoids are organized into concentric shells. See Figure 17.
Chlorophyll in Evolution
The emergence of organisms capable of harnessing energy from the sun to create their own food several million years ago is believed to have led to the emergence of the currently diverse photosynthetic species on Earth. A theory has been proposed suggesting that the ancient photosynthetic prokaryotes were taken as symbionts inside the larger cells of organisms. This form of symbiosis is referred to as “endosymbiosis”, which is presumed to have led to the emergence of eukaryotes. The larger cell (which eventually became the early eukaryote) engulfed a smaller cell (which is believed to be an early prokaryote) and eventually evolved into a semi-autonomous organelle. In particular, the smaller cell capable of performing photosynthesis eventually evolved into a chloroplast. This theory is called the Endosymbiotic theory.
The theory suggests that the eukaryotic cell took in the cyanobacteria as endosymbionts and led to the emergence of algal groups, such as Chlorophyta (belonging to green algae), Rhodophyta (red algae), and Glaucophyta. The chloroplasts of the Glaucophyta are of evolutionary importance because they have a peptidoglycan layer, which seems to be a ‘relic’ feature of the endosymbiont cyanobacterial ancestors. Chlorophyta (chlorophytes), in turn, possess similar chlorophylls as the land plants (embryophytes). Because the chlorophytes and the land plants have both chlorophylls a and b as their main photosynthetic pigments, they are believed to be ‘sister’ taxa. However, the land plants that we see today are presumed to have particularly descended from the ancestors of Charophyta (charophytes), which is another taxon belonging to the green algae.
The chlorophytes, the charophytes, and the embryophytes share common features, such as having chlorophyll a and b, cellulose in the cell wall, and storing starch as the food inside the plastids. Nevertheless, the charophytes and the embryophytes seem to be more closely related because they have more similarities than the chlorophytes. For example, both the charophytes and the embryophytes produce certain enzymes not found in the chlorophytes. Also, both the charophytes and the embryophytes use phragmoplasts in cell division (mitosis).
Try to answer the quiz below to check what you have learned so far about chlorophyll.
References
- Glagoleva, A. Y., Shoeva, O. Y., & Khlestkina, E. K. (2020). Melanin Pigment in Plants: Current Knowledge and Future Perspectives. Frontiers in Plant Science, 11. https://doi.org/10.3389/fpls.2020.00770
- Caventou, J.B. and Pelletier, P.J. (1817). “Notice sur la matière verte des feuilles” (Notice on the green material in leaves), Journal de Pharmacie, 3: 486-491. p. 490.
- Lange L, Nobel P, Osmond C, Ziegler H (1981). Physiological Plant Ecology I – Responses to the Physical Environment. 12A. Springer-Verlag. pp. 67, 259.
- Virtanen, O., Constantinidou, E., and Tyystjarvi, E. (2020). Chlorophyll does not reflect green light – how to correct a misconception. Journal of Biological Education. https://www.tandfonline.com/doi/full/10.1080/00219266.2020.1858930
- Hunt, R. (2004). The reproduction of colour. Chichester UK: Wiley–IS&T Series in Imaging Science and Technology. pp. 11–12. ISBN 0-470-02425-9.
- Lange L, Nobel P, Osmond C, Ziegler H (1981). Physiological Plant Ecology I – Responses to the Physical Environment. 12A. Springer-Verlag. pp. 67, 259.
©BiologyOnline.com. Content provided and moderated by Biology Online Editors.