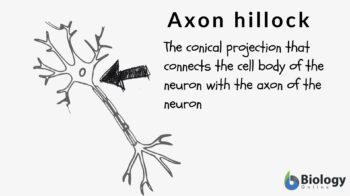
Axon hillock
n., plural: axon hillocks
[ˈæksɒn ˈhɪlək]
Definition: tapering region between a cell body and axon of a neuron
Table of Contents
Axon Hillock Definition
What is axon hillock? If you are familiar with the different parts of the neuron, the axon hillock is the conical projection of the neuron that connects the cell body (or soma) with the axon. In other words, the region where the axon is connected to the soma is at the axon hillock. So, this raises this question: Is the axon hillock part of the axon? Some references consider the axon hillock to be the originating point or the initial segment of the axon. Others see it as the base of the axon. Others still call this segment the trigger zone of neuron because the neuronal action potential is triggered and generated here.
If you are not familiar with a neuron and its parts, let’s have a short overview of the structure of a neuron.
Structure of Neuron
Neurons, or the nerve cells, are the differentiated cells of the nervous system responsible for distributing and receiving “signals” (impulses). Structurally, neuronal cells are unique from the rest of the body cells. Each neuronal cell has the following parts: cell body, axon, and dendrites. Let’s understand each of these neuronal parts.
Anatomy definition: Axon hillock is the neuronal projection that transmits the neuronal signals from the neuronal cell body to different body cells (Note: axons carry outward-bound signals) while the dendrites are the root-like projections that receive the signals from the other cells of the body and relay them to the nervous system (Note: dendrites carry inward-bound signals).
Medicine definition: Axon hillock is an axon nerve fiber that is a long projection of a neuron that carries the outbound neuronal cell signals as opposed to dendrites, which are the short protrusions from the neuronal cell body that brings in the inbound signals to the neuron.
Etymology: “axon”, from Greek “áxōn”, meaning “axle” or “axis”; hillock, from Middle English “hillok”, “hil”, meaning “hill”.
Let’s understand the difference between axons and dendrites (Table 1)
Table 1: Axon vs. Dendrites | |
---|---|
Axon | Dendrites |
One axon per neuron | Multiple dendrites per neuron |
Axon transmits the outgoing signals | Dendrites transmit the inbound or the incoming signals |
Relatively, axon are long ~sevral meter in length | Relatively smaller in length |
Axons possess a uniform thickness throughout their length, and the branching occurs only terminally | A highly branched structure that can be seen throughout its length |
Terminal ends of the axons form synaptic knobs | The tapering ends in dendrites; Absence of synaptic knobs |
Neurofibrils form the cytoskeletal structure of the axon. However, Nissl’s granules are absent | Presence of neurofibrils as well as Nissl’s granules |
Axons carry the signals away from the neuronal cell body | Dendrites carry the signals towards the neuronal cell body |
Axon location- between neuronal cell body or soma and the axon terminal | Dendrites location- multiple projections throughout the periphery of the neuronal cell body or soma. |
Axons are generally covered with a myelin sheath in order to preserve the signal during its transmission to different parts of the body. These axons are referred to as myelinated axons. However, some axons may be unmyelinated as well.
Depending upon the myelination of the nerve fibers, they can be classified into three types:
- Group A nerve fibers- myelinated axons
- Group B nerve fibers- myelinated axons
- Group C nerve fibers- unlike type A and B axons, type C axons are unmyelinated
Axons conduct a specific type of electric impulse, known as the action potential. These action potentials are outward-bound, i.e., from the neuronal cell body to the terminal end of the axons.
Sequential abrupt changes in the electric potential or the voltage across the plasma membrane are the action potential. Nonstimulated or resting axonal action potential is (−)60 mV. Take note that the negative sign indicates a relatively negative potential of the plasma membrane’s inner side to the outer side of the plasma membrane. This action potential of the neuronal cells is equivalent to the non-neuronal cells. This action potential can rise up to (+)50mV, which means that the inner action potential is positive relative to the plasma membrane’s outer side. Thus, a net change of ~110mV can occur during the depolarization of the axons.
This depolarization is followed by repolarization, i.e., returning to the non-stimulated or the resting action potential. The sequence of depolarization and repolarization of the axonal membrane results in the conduction or the transmission of the signals/action potential at a speed of 100 m/sec from the neuronal cell body to the different cells of the body.
The action potential moves along the axon toward the terminal part of the axon wherein at the chemical synapses it incites the release of neurotransmitters for further propagation of the signals.
The cell body is the core of the neurons that contain the genetic information, upholds the neuronal structure, and provides the energy for the neuronal activities. The nucleus and the other cellular organelles (like endoplasmic reticulum, Golgi apparatus, mitochondria, ribosomes, secretory vesicles, etc.) are present inside the cell body of the neurons. (Figure 1)
The bridge between the cell body and the axon is known as the axon hillock. The generation of the action potential occurs at the axon hillock. Most of the neurons possess one key axon and multiple dendrites. The terminal point of the axon is the presynaptic terminal (also referred to as terminal bouton). These terminal buttons connect with other neuronal cells to form a synapse. Thus, signals from one neuronal cell are transmitted to the other neuronal cell via these synapses.
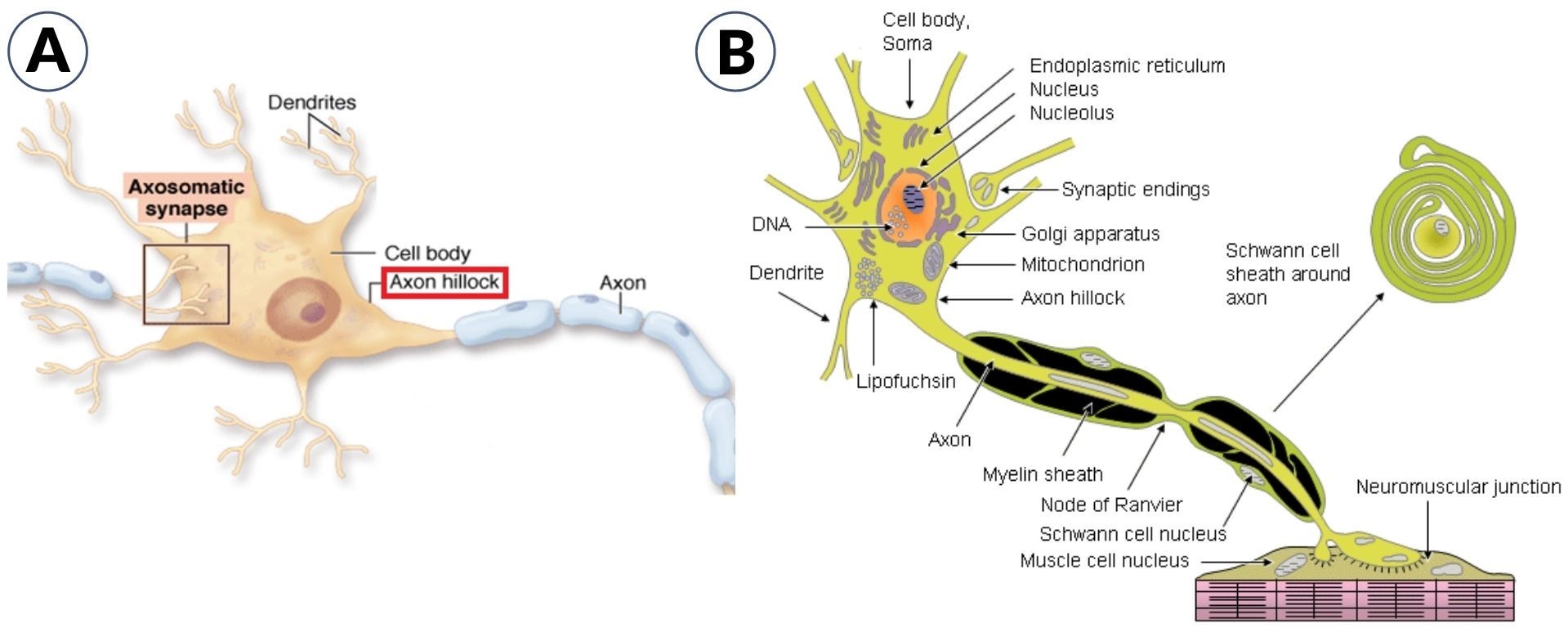
Now that we learned the basic parts of the neuron, let’s take a closer look at the axon hillock.
Axon Hillock Structure
Axon hillock is the neuronal region in the cell body wherein the summation of the excitatory & inhibitory activity occurs. The neuronal action potential is usually created at the axon hillock. It is a cone-shaped area in the neuronal cell body from where the axon originates. (Figure 1-A)
The initial region that lies between the axon hillock and the myelin sheath’s starting point is called the axon initial segment or AIS (Figure 2-A). The initial segment is the site for the initiation of the action potential. Characteristically, the axon hillock does not contain ribosomes and most of the other cell organelles. However, it does contain cytoskeletal elements or microtubular structures and organelles that move along the axon. (Figure 2-B) The neurofilaments in the axon hillock form a cluster, which is referred to as fascicles.
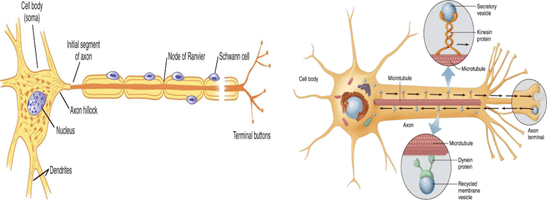
The terminal portion of the axon and its collaterals (i.e. collaterals are the branches of the central axon) form small branches or telodendria that form button-shaped tips known as terminal boutons (also called nerve endings or synaptic knobs) (Figure 3).
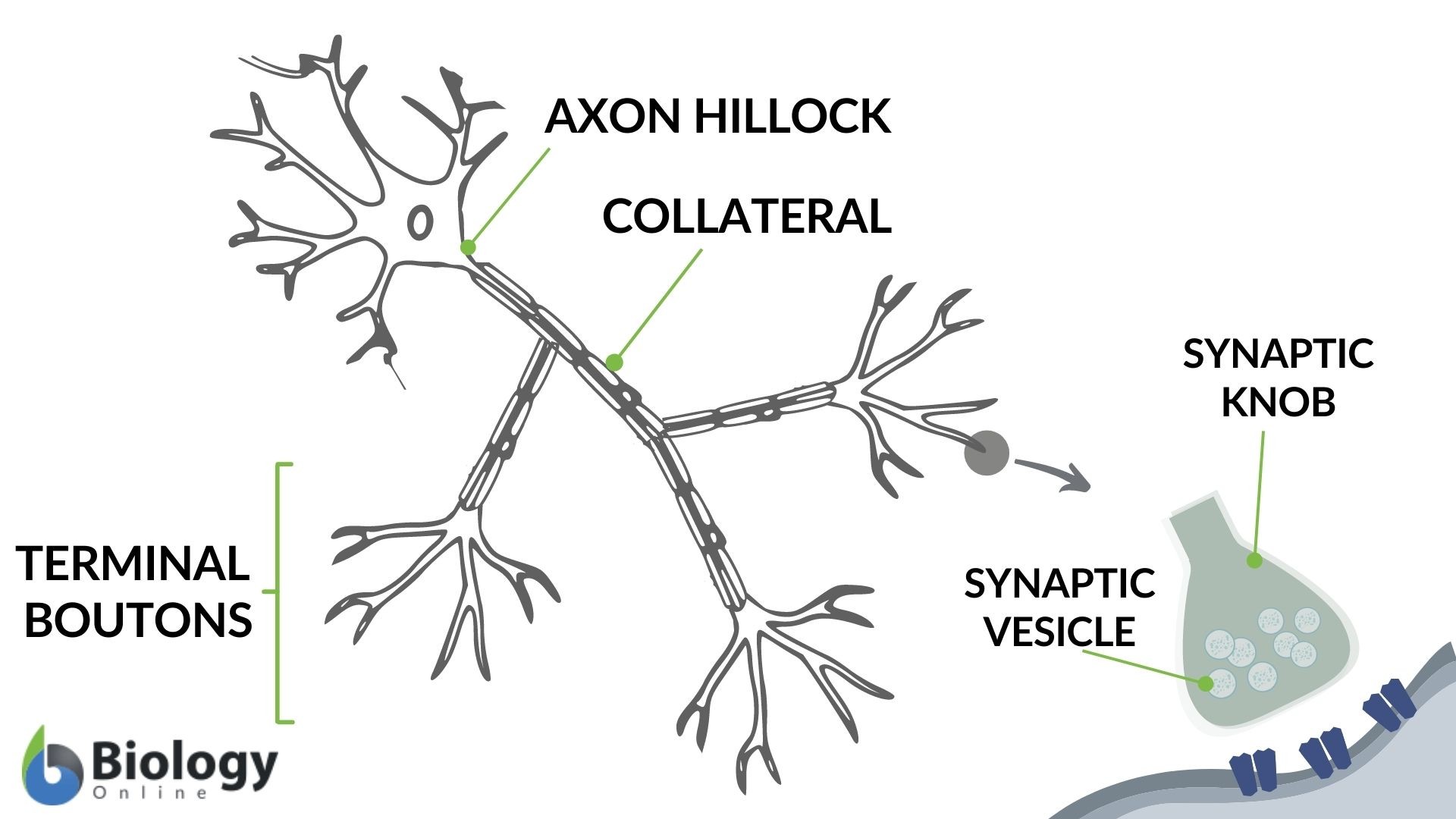
Another distinguishing feature of the axon hillock region in the neuron is the presence of a high density of voltage-gated sodium ion channels. The axon hillock region possesses almost 100-200/m2 of voltage-gated sodium ion channels, which are thought to be due to cytoskeletal and plasma-membrane-associated proteins, ankyrin.
Axon Hillock Function
What is the function of the axon hillock? Why does an action potential occur at the axon hillock?
The axon hillock acts as an administrator, sums up the total signals received, both inhibitory and excitatory signals. If this sum exceeds the limiting threshold, the action potential is triggered. This results in the transmission of the generated electrical signal through the axon away from the neuronal cell body. These signals are mediated through certain ion channels.
So, let’s understand: What type of ion channels are necessary for the function of the axon and the axon terminal?
This action potential is triggered by the alteration in the gated voltage sodium ion channels and the potassium ion channels that are sensitive to the changes in polarization.
On receiving the signals, sodium ions enter the cell, thereby, reducing the axonal polarization. In case this depolarization of the axon, due to the entry of sodium ion, crosses a specific threshold, an action potential is generated. This action potential will, then, be transmitted as an electrical signal through the axon to the synapses.
The generation of the action potential is an example of an all-or-none phenomenon, wherein either the neurons are fired or not, and the transmission of complete signal occurs (transmission of the partial signal never occurs).
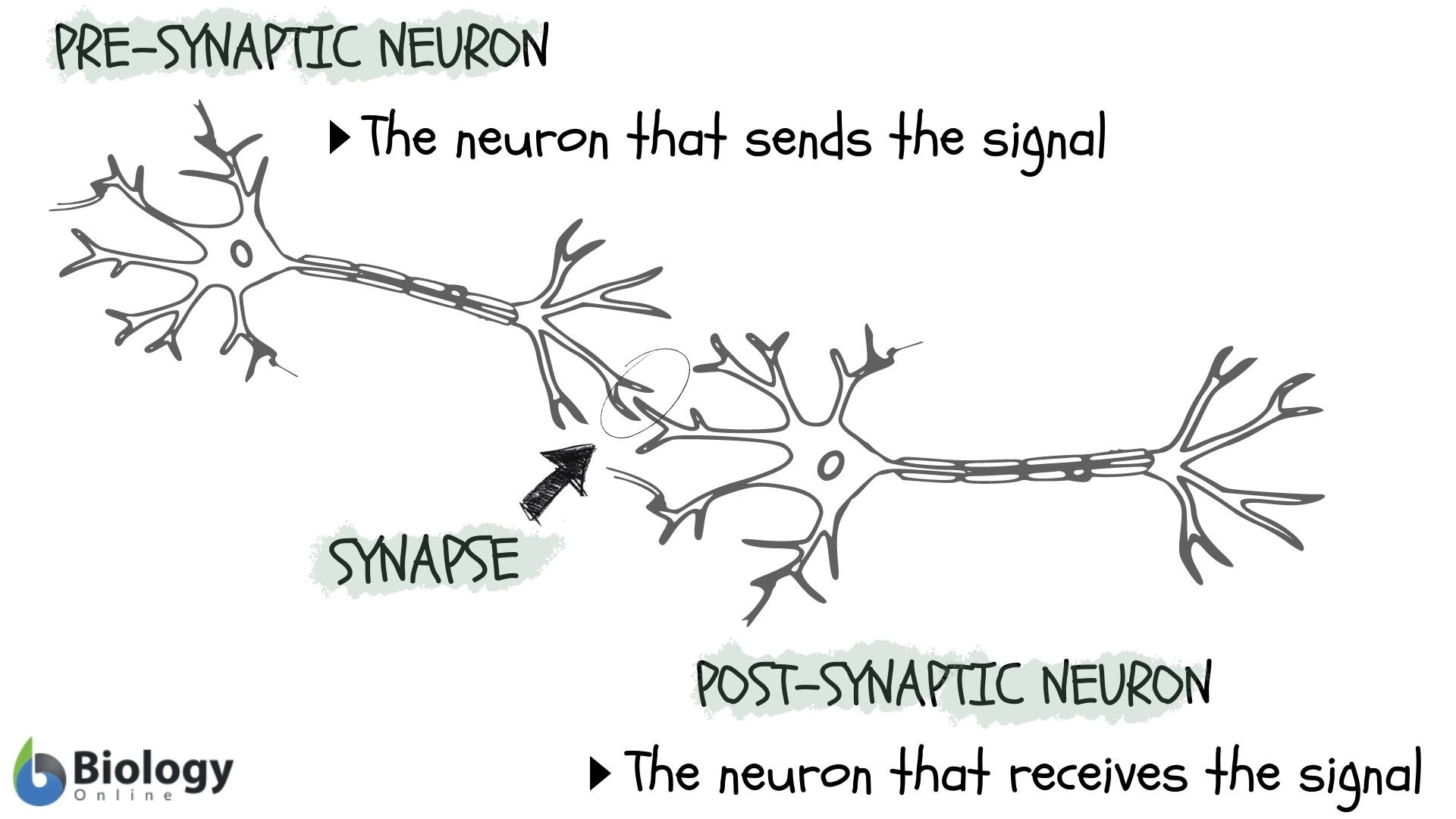
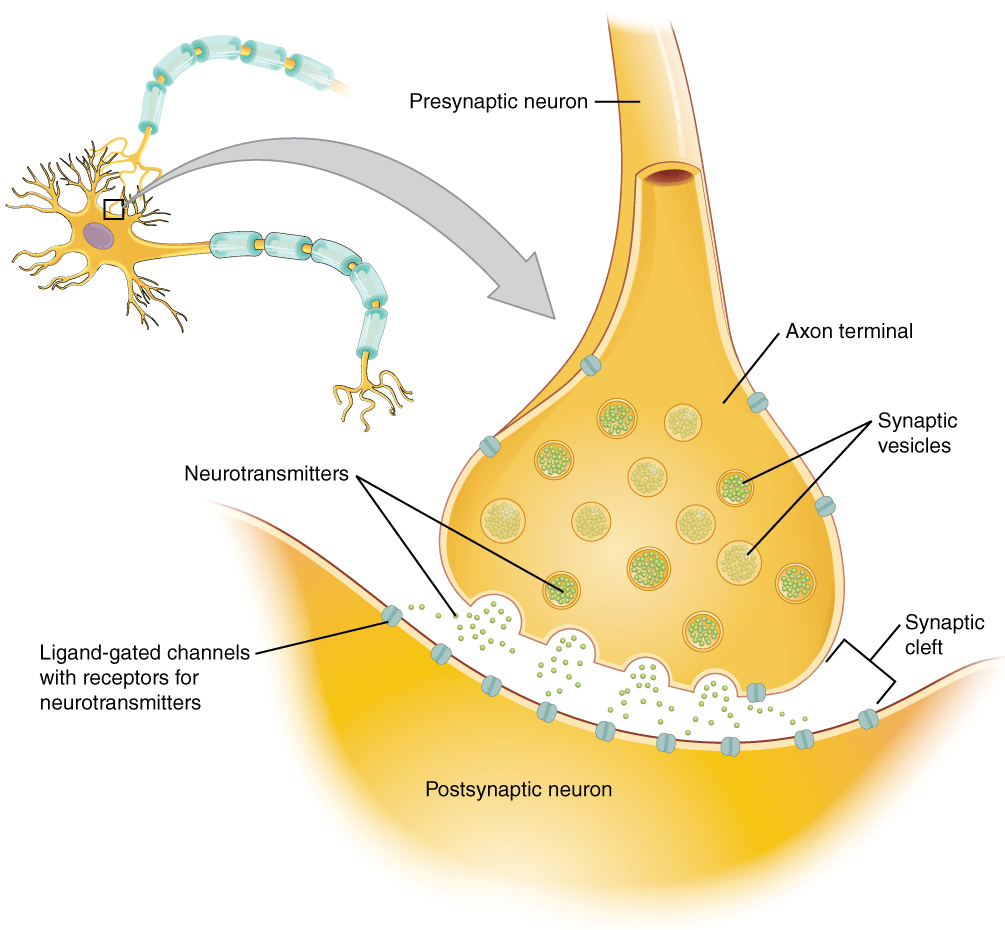
The whole process of action potential generation can be broadly divided into the following different stages (Figure 6):
- Rising phase and peak phase- At the threshold level, when the axon receives the signal, the axon hillock moves into the depolarization state that triggers the flow of external signals. This depolarization is also known as the rising phase. This phase is triggered by the sudden rush of positively charged sodium ions (Na+) through open voltage-gated sodium channels into a neuron. A further influx of the sodium ions reverses the polarity of the neuronal membrane and at this stage, the membrane develops a positive potential momentarily (+40 mV). This is the peak phase.
- Falling phase- Further, the voltage-gated sodium channels start to close slowly while voltage-gated potassium channels start opening up, resulting in the repolarization or falling phase. Consequently, the permeability of the neuronal membrane for sodium-ion starts to decline and reduce to resting level. Closing of the sodium ion channel results in the reduction of sodium ion entry; however potassium ions flux out due to opening of the voltage-gated potassium channels resulting in restoration of the localized negative membrane potential of the neuronal cell.
- Undershoot phase and refractory phase- Resetting of the sodium channels along with some open potassium ion channels results in the Hyperpolarization phase. Excessive efflux of the potassium ions occurs during this phase of hyperpolarization as potassium ion channels are open at this stage before these channels finally close. This is seen as a slight dip followed by a spike in the hyperpolarization phase.
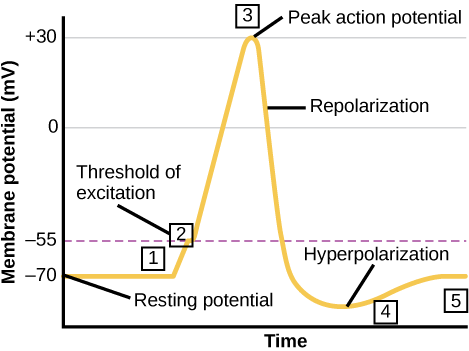
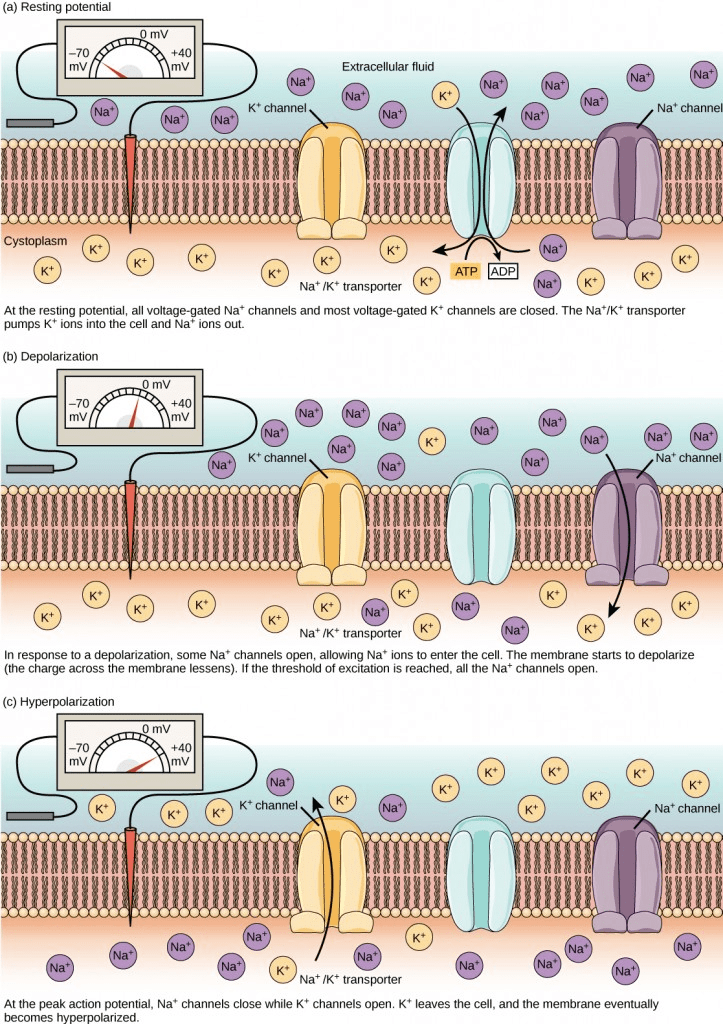
It is interesting to note that action potential always moves or transmits in one direction only, i.e., from the cell body or soma to the axonal synapse(s). Action potentials never go in reverse This is attributed to the presence of the voltage-gated sodium ion channels that once closed, remain closed for a period of 1-2 milliseconds, i.e., the refractory period. As a result, the refractory period forces the action potential to move only in one direction.
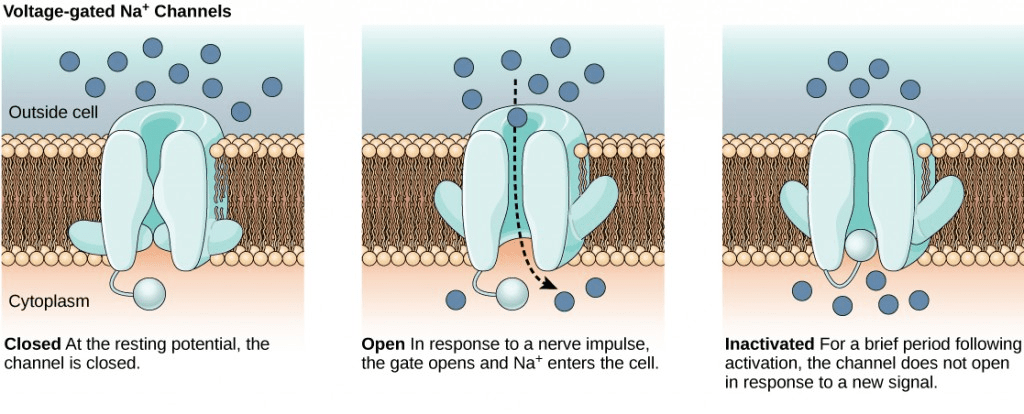
Axon Hillock Dysfunction
Many times, during depolarization/repolarization events, the positive sodium ions outside the cell membrane are not able to balance the ions that are present inside the cell, resulting in the generation of increased negative potential. This process is called hyperpolarization. During this stage, the positive ion channels don’t permit the entry and exit of ions. This creates neurotransmitters or voltage imbalance across the membrane resulting in various abnormalities ranging from a movement disorder, epileptic seizures, conversion disorder, and other neural abnormalities.
Axon hillock and axon initial segment are the key regions for the generation of action in the neurons. It has been found that gene mutations that result in alteration of the genetic constitution of axon initial segment or any disturbances in the molecular organization of the axon initial segment or any changes in the axon initial segment ion channel expression/function/ location and/or density are responsible for the various neurological disorders.
Recent findings suggest that many genes that encode for the proteins found at the axon initial segment are emergent key risk factors for neurological diseases like epilepsy, schizophrenia, bipolar disorder, etc.
Cognitive dysfunction in patients with schizophrenia has been ascribed to the disruption in the GABAergic circuit. More precisely, it has been found that in patients with schizophrenia, immunoreactivity of the GABAA receptor α2 subunit is increased at the post-synaptic membrane of the axon initial segment. This has further been attributed to the mutation in the ANK3 gene that encodes for the Ankyrin 3 protein.
Bipolar disorder has also been found to be related to the ANK3 gene.
Another neurological disorder related to dysfunctional axon hillock is Autism spectrum disorders. Autism spectrum disorder is characterized by cognitive dysfunction of varying severity. In autism, a genetic mutation in the CNTNAP2 gene results in impaired synaptic development, a function that results in synaptic plasticity. This synaptic plasticity eventually results in altered sodium ion channel expression and axon initial segment ion channel density, which results in cognitive dysfunction.
Trauma-like ischemia-induced neuronal injury following a stroke incidence can result in calpain-mediated disassembly of the cytoskeleton of the axon initial segment. Breakdown of the cytoskeleton results in loss of voltage-gated sodium ion channel cluster. This results in neuronal death.
Neuronal hyperexcitability in the central nervous system has been found to be associated with epilepsy, insomnia, and short-term memory loss. Serum antibody profile of the patients of several autoimmune disorders like multiple sclerosis, Morvan syndrome, etc., indicates the involvement of genetic mutations pertaining to axon hillock and axon initial segment dysfunction.
The voltage-gated sodium channels found in axon hillock and axon initial segment are made up of a single pore-forming α subunit and two auxiliary β subunits. Genetic mutations in α and β subunits of voltage-gated sodium ion channels result in hyperexcitability and seizure activity. Dravet syndrome or severe myoclonic epilepsy in infancy has been found to be due to the loss of functional sodium ion voltage channels.
Thus, axon hillock and axon initial segment are the neuronal regulators, and dysfunctional axon hillock may result in numerous neurological disorders.
Test your understanding of the axon hillock by answering the quiz below.
References
- Muzio MR, Cascella M. Histology, Axon. [Updated 2020 May 26]. In: StatPearls [Internet]. Treasure Island (FL): StatPearls Publishing; 2021 Jan-. Available from: https://www.ncbi.nlm.nih.gov/books/NBK554388/
- Wimmer, V. C., Reid, C. A., So, E. Y., Berkovic, S. F., & Petrou, S. (2010). Axon initial segment dysfunction in epilepsy. The Journal of physiology, 588(Pt 11), 1829–1840. https://doi.org/10.1113/jphysiol.2010.188417
- Buffington, S. A., & Rasband, M. N. (2011). The axon initial segment in nervous system disease and injury. The European journal of neuroscience, 34(10), 1609–1619. https://doi.org/10.1111/j.1460-9568.2011.07875.x
- Leterrier C. (2018). The Axon Initial Segment: An Updated Viewpoint. The Journal of neuroscience : the official journal of the Society for Neuroscience, 38(9), 2135–2145. https://doi.org/10.1523/JNEUROSCI.1922-17.2018
©BiologyOnline.com. Content provided and moderated by Biology Online Editors.