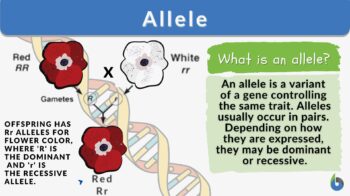
Allele
n., plural: alleles
/ˈæ.liː.l/
Definition: variant of a gene
Table of Contents
Allele Definition
What are alleles? An allele is a term coined to describe a specific copy of a gene. Genes, the DNA sequences controlling our traits, are usually found in two copies in eukaryotic genomes; each copy (allele) is inherited from one parent. Each allele occupies a specific region on the chromosome called a gene locus. The gene’s two alleles are located in the same region in two homologous chromosomes, one inherited from each parent. The alleles may be dominant or recessive. The dominant allele (definition: the allele that is expressed) masks the effect of the recessive allele (definition: the allele that is not expressed).
READ: What is dominance? Examples and Punnet Square – Biology Online Tutorial
The term “phenotype” is used to describe a visible trait or characteristic. Assuming that the trait is controlled by only one gene, both alleles constitute a genotype. Accordingly, the term genotype is used to describe an organism’s set of alleles coding for each trait. Depending on each allele’s DNA sequence, the genotype can be described as homozygous or heterozygous of a particular gene. Homozygous genotype means that the organism’s genome has two identical alleles for a specific gene. That means both alleles contribute equally to the appearance of the trait. Heterozygous genotype, in contrast, contains two different forms of a particular gene.
There are genes, however, that have more than two alternative allelic forms, they are referred to as multiple alleles.
Allele Examples
Below are examples of alleles and how they are expressed. The allelic expression may follow a Mendelian pattern of inheritance — as in the case of complete dominance. As for nonMendelian inheritance, the alleles may be expressed through codominance, incomplete dominance, or polygenic inheritance.
Complete dominance
One classic example explaining how alleles work is the alleles responsible for the flower color in garden peas, one of the seven traits studied by Gregor Mendel in his experiments that later established the principles of genetics.
The color of garden peas flowers can be either purple or white. The gene coding for this trait, responsible for producing a purple pigment, is found in two forms, allele for purple color and allele for white color.
Alleles are designated by letters to describe genotypes. The purple color’s allele (referred to as P) can naturally express the purple pigment while the white color allele (referred to as p) cannot. Hence, the resulting genotype is written as Pp. Other genotypes that can be found for this trait are PP (Purple flower) and pp (white flower). However, one P allele can produce enough pigment to make the flower purple color in this particular example. Therefore, plants with either PP or Pp genotypes will have purple flowers, and only plants with the pp genotype have white flowers.
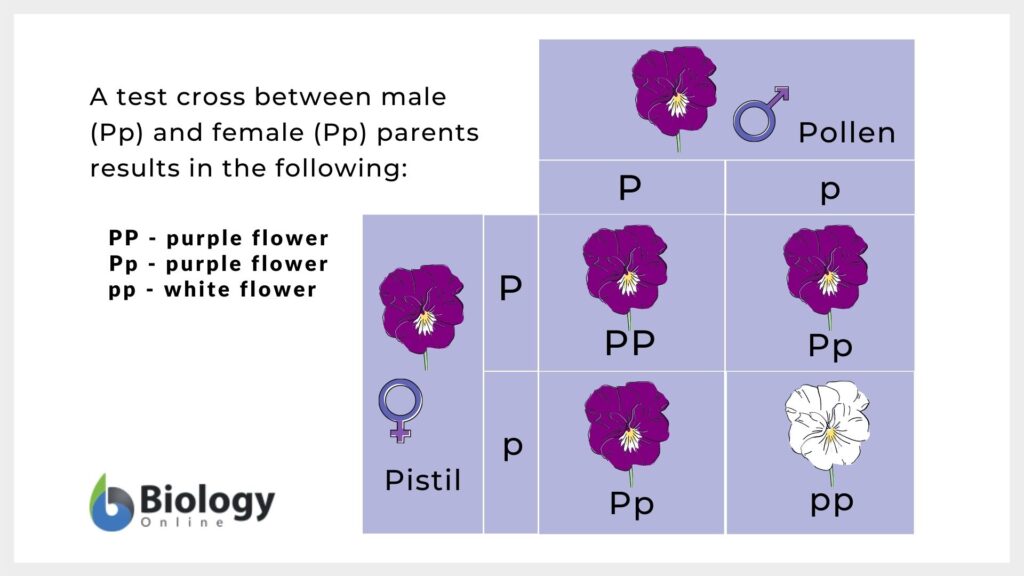
In this situation, the allele that expresses the trait over the other trait is called the dominant allele (the P allele in this case). The other allele (p) is called recessive and is only expressed when present in two copies.
However, this is not always the case. In some genes, two different alleles (heterozygous genotype) are equally expressed, a phenomenon called codominance. One of the most common examples of this type of inheritance is ABO blood grouping alleles.
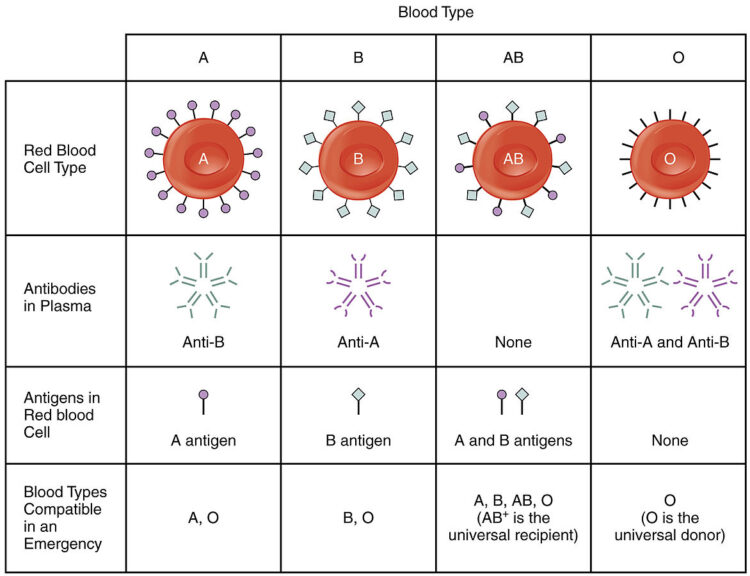
ABO – Codominance
ABO locus has three allelic forms: A, B, O. The genotype of this gene determines the antigens on the red blood cells, hence the blood group phenotype. Both A and B alleles encode for glycosyltransferase which catalyzes the final step in the A and B antigens’ synthesis pathway, while the O allele codes for an inactive version of the enzyme. The enzymes coded by A and B alleles differ in only four amino acids, due to several single nucleotide polymorphisms (SNPs) in the ABO gene.
The table below shows the inheritance pattern of the ABO gene. Since the locus has three alternative alleles, six possible genotypes can result from mating. However, only four phenotypes (blood groups) occur, A, B, AB, and O. Two inheritance patterns can be seen in blood groups. Both A and B alleles are dominant when they are found with the O allele. However, If a person has an AB genotype, both alleles are equally expressed (codominance), resulting in a phenotype where both A and B antigens are found on the surface of red blood cells.
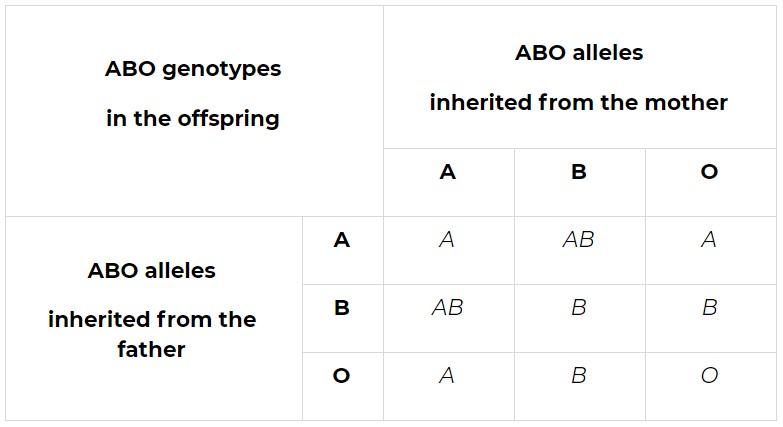
Multiple alleles
Some genes could have more than two alternative allelic forms. Many know human genes have multiple alleles. Each individual can have only two alleles of each gene. However, some genes could have hundreds of alleles within a population. Any allele results from a change in a gene’s DNA sequence, even if it occurs in a single nucleotide. Therefore, multiple alleles of a given gene do not necessarily mean variation in the phenotype. Many allelic forms result from changes in the gene’s sequence without affecting protein sequence or function. All these ‘normal’ alleles can be described as “wild-type” alleles compared to mutant alleles that affect protein structure or function.
Multiple alleles are also used to describe all mutations found in a gene in a population. For example, multiple loss-of-function mutations can be reported in a population of patients suffering from a genetic disease. Although different mutations lead to the same result, loss of protein function, each mutation constitutes a different mutant allele as long as it is located in a different gene site.
Beta-thalassemia and β-globin gene
Beta-thalassemia is characterized by the reduction or absence of beta-globin chains of the hemoglobin. More than 300 β-thalassemia alleles have been reported so far; most of them are inherited in a Mendelian recessive pattern. Most mutations that were reported involved a single or a few nucleotides within the β-globin gene or its flanking regions. Some deletion mutations have also been reported and result in the complete loss of β chain production. The severity of the disease depends mainly on the quantitative reduction level of β chains. Due to the wide range of mutations reported in the β-globin gene and the variation of phenotypes observed, Beta-thalassemias are observed as a group of inherited blood disorders.
STR alleles and forensic identification
Short tandem repeats (STRs) are short repetitive DNA sequences (2-6 nucleotides) scattered all over the genome. They are non-coding DNA sequences but can be linked to specific genetic conditions. However, the primary and most successful application of STR markers is forensic analysis. The number of repeats in each STR locus is highly variable between individuals. Scientists used these variations within a population and developed a test for forensic identification using multiple STR markers. The use of multiple markers in the same assay gives a high power of discrimination in the form of a unique “DNA fingerprint” that can be used for human identification in cases like crime suspects or unrecognized bodies.
Allelic Variation
Going back to the discussion of the ABO blood groups, genetic variation between alleles could lead to a protein variation. Even a small variation in protein level, four amino acids, in this case, can result in drastic effects on the phenotypes. Indeed, a blood transfusion to an incompatible patient could lead to death due to eliciting the recipient’s immune response to the ABO antigens present on the donor’s RBCs. Therefore, it can be said that one of the significant factors contributing to the genetic variation observed between individuals is the allelic variation between their genes.
Discontinuous variation
The blood group and flower color phenotypes are examples of discontinuous variation, where a trait is found in two or more distinct alternative forms. In this type of variation, the different phenotypes can be easily distinguished. Geneticists use the term polymorphism to describe the traits with two or more common phenotypes in a population and morphs to describe the individual phenotypes. In some cases, rare, exceptional phenotypes occur; these are called mutants, and the more common normal phenotype is called wild-type. Although both polymorphisms and mutations originate from DNA sequence changes, somehow, polymorphism changes became more common.
Continuous variation
The second type of genetic variation is called continuous variation. In this type of variation, a trait shows a continuous range of phenotypes that cannot be distinguished as distinct phenotypes, as is the case with a discontinuous variation. Examples of characters that show continuous variation include weight, height, eye color, and similar measurable characteristics. Such traits are usually encoded by more than one gene; analyzing these phenotypes is more complicated than those showing discontinuous variation.
Molecular basis of allelic variation
Phenotypes can be expressed due to a single gene’s activity, as in most discontinuous phenotypes, or several genes, as in continuous phenotypes. Most genes code for proteins that directly express the trait. Being the direct product of gene expression, proteins are the main factors presenting the phenotype by executing their cellular functions. Proteins can be receptors responsible for presenting antigens to the immune cells, pigments, hormones, antibodies, or enzymes.
Genetic variation in the genes code for these proteins can have several consequences, ranging from no effect to disrupting these genes’ function. Some genetic variations involve the change of a single nucleotide, the building block of DNA. Such mutation, referred to as nucleotide substitution, is the most common and simplest form of genetic variation. If a substitution occurs in a coding region within the gene, that will result in amino acid substitution.
The effect of amino acid substitution on the protein function and the phenotype depends primarily on the changed amino acid site within the protein’s three-dimensional structure. For example, the function of an enzyme is to catalyze chemical reactions by binding to its substrates through an active site, a region within the 3D structure of the enzyme that is specifically bound to a substrate in a lock-and-key manner. And since biological function depends on structure, any slight change in the active site’s 3D structure will directly affect the enzymatic function. Therefore, a base-pair substitution that results in changing an amino acid in the active site or in a different region that will change the active site’s 3D structure will prevent enzyme activity. Other base substitutions can create a stop codon resulting in the premature termination of translation and the creation of truncated protein products.
Mutations can also occur in the form of an addition or deletion of one or a few nucleotides. The effect of these mutations is usually more damaging to the protein because the mRNA molecule is read in a group of three “codons”; therefore, unless the deletion or insertion involves a multiple of three nucleotides, a shift in the reading frame will result, changing all the subsequence codons. Such mutations are called “frameshift mutations.”
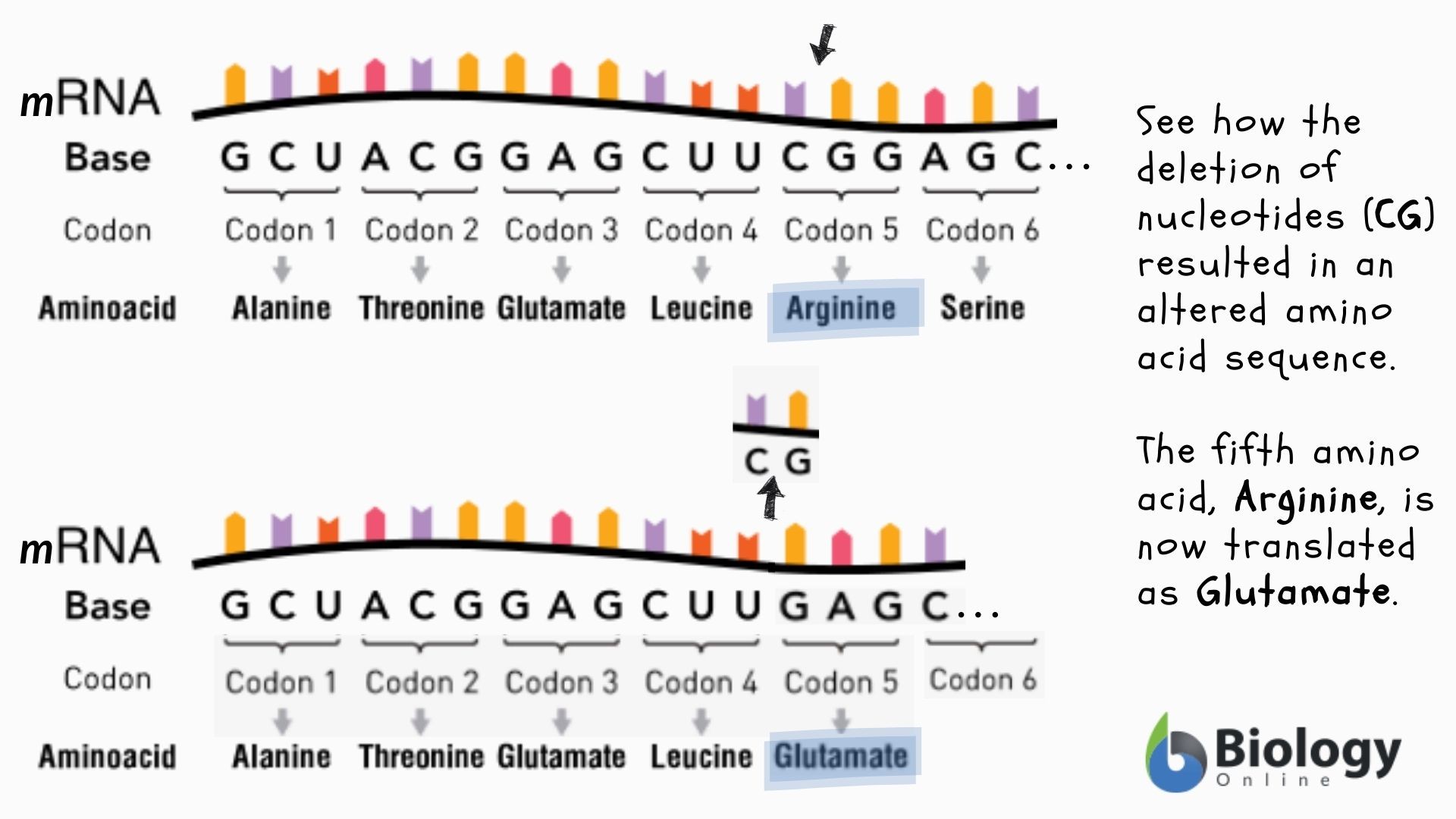
You may view this video below to understand further the alleles and genes.
Try to answer the quiz below to check what you have learned so far about alleles.
References
- Dean L. Blood Groups and Red Cell Antigens [Internet]. Bethesda (MD): National Center for Biotechnology Information (US); 2005. Chapter 5, The ABO blood group. Available from: https://www.ncbi.nlm.nih.gov/books/NBK2267/
- Hartl, D. L. (1998). Genetics: principles and analysis.
- Klug, W. S., & Cummings, M. R. (2006). Concepts of genetics. Upper Saddle River, NJ: Pearson Education,.
- Wyner, N., Barash, M., & McNevin, D. (2020). Forensic Autosomal Short Tandem Repeats and Their Potential Association With Phenotype. Frontiers in genetics, 11, 884.
- Thein, S. L. (2013). The molecular basis of β-thalassemia. Cold Spring Harbor perspectives in medicine, 3(5), a011700.
©BiologyOnline.com. Content provided and moderated by BiologyOnline Editors.